Neuronal regeneration in the goldfish telencephalon following 1-methyl-4-phenyl-1,2,3,6-tetrahydropyridine (MPTP) insult
Abstract
The constitutive regenerative ability of the goldfish central nervous system makes them an excellent model organism to study neurogenesis. Intraperitoneal injection of neurotoxin 1-methyl-4-phenyl-1,2,3,6-tetrahydropyridine (MPTP) was used to deplete tyrosine hydroxylase-positive neurons in the adult goldfish telencephalon. We report novel information on the ability of the goldfish to regenerate (∼3–4 d post-MPTP insult) damaged neurons in telencephalic tissue by observing the rapid incorporation of bromodeoxyuridine into newly generated cells, which precedes the recovery of motor function in MPTP-treated animals. Specifically, the telencephalon area telencephali pars dorsalis in female goldfish, which is associated with fish motor activity, regenerates following MPTP toxicity. The remarkable ability of goldfish to rapidly regenerate damaged neurons provides insight into their use as model organisms to study neuroregenerative abilities within a few days following injury. We provide evidence that goldfish are able to regenerate neurons in ∼3–4 d to both replenish and recover baseline catecholaminergic levels, thus enabling the fish to reestablish basic activities such as swimming. The study of neuron regeneration in the damaged goldfish brain will increase our understanding of vertebrate neurogenesis and regeneration processes following central nervous system injury.
Introduction
Goldfish (Carassius auratus (Linnaeus, 1758)) and other teleosts are amenable model organisms to study neurogenesis (Stevenson and Yoon 1978; Stuermer et al. 1992; Sullivan et al. 1997) because they exhibit remarkably higher neurogenic rates than mammals and, thus, a greater ability to regenerate damaged axons and tissues following central nervous system (CNS) injury (Levine 1983; Stuermer 1986). It has been estimated that the rate of neurogenesis in the CNS of teleosts is approximately one order of magnitude higher than that observed in mammals (Zupanc and Sîrbulescu 2011). For example, ∼6000 cells or 0.06% of the total zebrafish (Danio rerio (Hamilton, 1822)) brain cell population are newly generated within any 30 min period (Hinsch and Zupanc 2007; Zupanc and Sîrbulescu 2011). Newly generated neurons originate from radial glial cells (RGCs), which are known as one of the major progenitor cell populations (Pellegrini et al. 2007; Ganz et al. 2010; Chapouton et al. 2011; Rothenaigner et al. 2011; Xing et al. 2014). There are up to 16 proliferative zones in the teleost brain and the telencephalon exhibits numerous cellular pools and is a major source of RGCs (Zupanc and Horschke 1995; Zupanc et al. 2005; Kaslin et al. 2009; März et al. 2010; Pellegrini et al. 2016). In this study, we provide evidence that female goldfish can regenerate neurons ∼3–4 d post central nervous system injury leading to the recovery of cathecolaminergic baseline levels to re-establish normal basic functions such as swimming activities. Only female goldfish were used in this study as differences in cellular proliferation and neurogenesis in adult fish exists between sexes (Zikopoulos et al. 2000; Ampatzis and Dermon 2007).
The neurotoxin, 1-methyl-4-phenyl-1,2,3,6-tetrahydropyridine (MPTP), can destroy dopaminergic neurons in the brain of teleosts and induce dopamine (DA) depletion and Parkinson-like symptoms (Poli et al. 1990; Pollard et al. 1992; Youdim et al. 1992; Lucchi et al. 1998; Lam et al. 2005; McKinley et al. 2005). The MPTP models of Parkinson’s disease (PD) experience many of the symptoms exhibited by human patients. This includes neuronal cell body destruction in the CNS motor axis as well as decreased tyrosine hydroxylase (TH) activity and DA content in the forebrain, midbrain, cerebellum, and vagal lobes of fish (Poli et al. 1990; Pollard et al. 1996), mice (Hallman et al. 1985), rat (Chiueh et al. 1984), guinea pigs (Chiueh et al. 1984), and primates (Burns et al. 1983). The goldfish ventral telencephalon, in particular the area telencephali pars dorsalis (Vd), plays a role in motor control in teleosts and is a region negatively impacted by MPTP toxicity (Goping et al. 1995; Wullimann and Mueller 2004; Sallinen et al. 2009). The ability of fish to regenerate damaged cells and tissues and reestablish normal functions following injury has been extensively studied in the spinal cord (Bernstein 1964; Sîrbulescu et al. 2009), cerebellum (Adolf et al. 2006; Zupanc and Sîrbulescu 2011), and retina (Stuermer et al. 1992; Becker and Becker 2007, 2008). Previous studies have reported reversible changes in forebrain DA levels following MPTP treatment where DA returned to baseline levels ∼8 d post-injection (dpi) (Pollard et al. 1992; Adeyemo et al. 1993; Popesku 2009).
The ability of fish to incorporate bromodeoxyuridine (BrdU) into mitotically active cells following injury has been reported by previous studies (Zupanc and Horschke 1995; Ampatzis and Dermon 2007; Zupanc and Sîrbulescu 2011). For example, actively dividing cells were visualized 1–10 d following a lesion in the cerebellum of the weakly electric fish Apteronotus leptorhynchus (Ellis in Eigenmann, 1912) (Zupanc and Ott 1999). In this animal model, newly generated cells were able to migrate to specific target areas, differentiate into neurons, and integrate into existing neural networks (Stroh and Zupanc 1996; Zupanc and Ott 1999). Zupanc and Horschke (1995) showed that BrdU is available for DNA synthesis 4 h after intraperitoneal injection in A. leptorhynchus and another electric fish, a species of Eigenmannia (Jordan and Evermann, 1896), and is able to incorporate into new cells and mark the occurrence of neurogenesis.
Teleost species remove damaged or compromised cells almost exclusively via apoptosis following CNS injury in comparison with necrotic cell death observed in mammalian models (Beattie et al. 2000; Vajda 2002; Liou et al. 2003). There is also activation of RGCs in the goldfish hypothalamus and telencephalon following MPTP administration marked by the observed increase of glial fibrillary acidic protein (GFAP) (Xing et al. 2017). The role of glial activation following MPTP administration in teleosts is not well understood but could potentially contribute to the generation of newly synthesized neurons in the goldfish brain.
In this study, we report on the ability of the goldfish to rapidly regenerate (∼3–4 dpi) damaged DAergic neurons in the area Vd by observing the rapid incorporation of BrdU into newly generated cells, which precedes recovery of motor function in MPTP-treated animals. The tyrosine hydroxylase (TH) immunoreactivity was used to determine if new catecholaminergic neurons are generated after MPTP administration. The rapid neuroregenerative ability observed in the goldfish telencephalon provides an explanation for the reported recovery of motor function and catecholaminergic levels in the goldfish brain following CNS injury.
Materials and methods
Animal maintenance
Adult female goldfish (Carassius auratus (Linnaeus, 1758)) were purchased from a commercial supplier (Mt. Parnell Fisheries Inc., Mercersburg, Pennsylvania, USA) and maintained at 18 °C under a natural-simulated photoperiod on standard flaked goldfish food. Fish were kept in 70 L tanks (15–18 fish per tank). All procedures were performed according to the guidelines of the Canadian Council on Animal Care and were approved by the University of Ottawa animal care committee. Goldfish were anesthetized using 3-aminobenzoic acid ethylester (MS-222; 0.05% in water, Sigma-Aldrich, Oakville, Ontario, Canada) for all handling and sampling procedures.
Experimental and sampling procedures
The neurotoxin MPTP (Sigma-Aldrich M0896, Oakville, Ontario, Canada) was dissolved in 0.6% saline to give a dose of 50 μg/g body weight of fish at 2 μL/g. Sexually recrudescent female goldfish (September 2014 experiment; ∼33 g ± 1.6; gonadosomatic index ∼2.8 ± 0.5) either received a single intraperitoneal injection of MPTP or a control 0.6% saline injection at time 0 similar to the treatment schedule used by Pollard’s group (Pollard et al. 1992; Goping et al. 1995). All female goldfish also received a single intraperitoneal injection of BrdU (Life Techonologies B23151, Burlington, Ontario, Canada) dissolved in 0.6% saline at a dose of 150 μg/g body weight at 3 dpi of MPTP prior to sampling at 4 and 7 dpi. Female goldfish were randomly sampled at 4 and 7 dpi when they were perfused intracardially with 0.6% saline (∼10 mL) and then 4% paraformaldehyde (PFA, Sigma-Aldrich, P6148, Oakville, Ontario, Canada) freshly depolymerized in phosphate-buffered saline (PBS; pH = 7.4). Following perfusion, the brains, whole pituitary, and gonads were carefully dissected from the goldfish. The gonadosomatic index was calculated by dividing the gonad weight by body weight of the goldfish × 100.
Behavioural analysis
Female goldfish (December 2014 experiment; 32 g ± 1.4; gonadosomatic index 1 ± 0.1; n = 10) received either a single intraperitoneal injection of MPTP (50 μg/g body weight) or a control 0.6% saline injection at time 0. The mobility measurements were performed once per day at 0, 1, 3, and 7 dpi in the late morning where time 0 was measured immediately after the administration of MPTP. Fish movement and swimming motions were recorded using an Olympus Stylus TG-850 (Olympus, Center Valley, Pennsylvania, USA) camera in individual tanks. The fish were placed in 4 cm of water in a 13 cm × 30 cm transparent aquarium similar to the experiment performed by Weinreb and Youdim (2007). The fish were allowed to acclimatize for 30 min prior to recordings. The total movement of a single fish, which includes the total distance of swimming and time of rest, was measured over a period of 5 min using Logger Pro 3 (Vernier Software & Technology, Beaverton, Oregon, USA). Statistical analysis was performed using SPSS Statistics (version 21, IBM, New York, New York, USA). The data for total distance traveled were normally distributed and compared using one-way analysis of variance (ANOVA) followed by Tukey’s test to compare the effects of MPTP treatment. The resting time data were not normally distributed and were therefore compared using the Kruskal–Wallis test followed by Dunn’s multiple comparison test to determine the effects of MPTP treatment. p-values <0.05 were considered statistically significant.
Immunostaining
Goldfish brains were dissected and collected after intracardial perfusion and postfixed with 4% PFA overnight at 4 °C. The following day, the brains were washed in a series of solutions (1× PBS (2× 30 min); 0.85% NaCl (1× 40 min); Ethanol (EtOH) 70%/NaCl 0.85% 1:1 (1× 30 min); EtOH 70% 2× 20 min; EtOH 85% (1× 40 min); EtOH 95% (1× 40 min); EtOH 100% (2× 30 min)) at 4 °C in preparation for paraffin embedding (toluene (2× 45 min); melted paraffin (3× 60 min)). All the brains were embedded in paraffin prior to sectioning. Six-micrometre sections were obtained for staining using a motorized microtome (HM 350, Microm, Heidelberg, Germany) and the sections were placed on Superfrost plus microscopic glass slides (Fisher Scientific 12-550-15, Toronto, Ontario, Canada). To visualize incorporated BrdU and TH, the paraffin sections were deparaffinized by washes in Xilol (2× 20 min) and serial dilutions of ethanol (2 min per wash) (2× EtOH 100%; EtOH 95%; EtOH 85%; EtOH 70%; EtOH 50%; EtOH 30%). The sections were then washed with 1× PBS (2× 10 min) prior to incubation with 50% formamide/50% 2× saline–sodium citrate (SSC) for 3 h in a 65 °C water bath. Following the incubation, the sections were washed with 2× SSC (20× SSC stock solution: 3 mol/L NaCl, 0.3 mol/L Sodium citrate, pH 7.0) (2× 5 min) prior to DNA denaturation by incubating the sections in 2 N HCl for 30 min at 37 °C. This was followed by washing in sodium borate buffer (0.1 mol/L, pH 8.5) for 2× 5 min at room temperature (RT) and then with 1× PBS with 0.2% TritonX-100 (3× 5 min). Nonspecific protein binding sites were blocked using 1% nonfat milk with 0.2% Triton X-100 in 1× PBS for 45 min at RT. Sections were then incubated with rat anti-BrdU (dilution 1:300; Abcam ab6326, Cambridge, Massachusetts, USA) and rabbit anti-TH antibodies (dilution 1:400; Millipore Ab152, Toronto, Ontario, Canada) in 1% milk with 0.2% Triton X-100 in 1× PBS at 4 °C overnight in a moist chamber. After 1× PBS with 0.2% TritonX-100 rinses of 3× 10 min on a shaker, the sections were incubated in Alexa Fluor 594 goat anti-rat immunoglobulin G (IgG) (dilution 1:300; Invitrogen A11007, Burlington, Ontario, Canada) and Alexa Fluor 488 goat anti-rabbit IgG (dilution 1:300; Life Technologies A11008, Burlington, Ontario, Canada) secondary antibodies in 1× PBS with 0.2% TritonX-100 for 1.5 h at RT. The sections were then washed three times in 1× PBS with 0.2% Triton X-100 for 5 min and mounted with anti-fading medium Vectashield with 4,6-diamino-2-phenylindole (DAPI) (Vector Laboratories, Burlingame, California, USA) and kept in the dark until visualization of slides with Nikon A1RsiMP confocal (Nikon, New York, New York, USA) microscope.
Immunocytochemistry for GFAP was used to identify RGCs (Forlano et al. 2001; Xing et al. 2015) in relation to TH-immunoreactivity in the ventral telencephalon. The paraffin sections were deparaffinized as described above and then washed with 1× PBS (2× 10 min). Nonspecific protein binding sites were blocked with 1% milk with 0.2% Triton X-100 in 1× PBS for 45 min at RT. Sections were then covered with mouse anti-pig GFAP antibody (1:500; Chemicon, Temecula, California, USA, Cat. # MAB360), which was previously validated in fish (Forlano et al. 2001; Xing et al. 2017) and rabbit anti-rat TH antibody (1:500; Chemicon, Tamecula, California, USA, Cat. # AB152), which was previously validated in fish (Yamamoto et al. 2011; Xing et al. 2017) overnight at 4 °C. Sections were then washed for 2× 10 min in PBS and incubated with a donkey anti-rabbit Alexa fluor 488 (1:500; Invitrogen Molecular Probes, Invitrogen, Burlington, Ontario Canada) and goat anti-mouse Alexa fluor 596 (1:500; Invitrogen Molecular Probes, Invitrogen, Burlington, Ontario Canada) for 1 h at RT. The sections were then washed three times in 1× PBS with 0.2% Triton X-100 for 5 min and mounted with antifading medium Vectashield with DAPI (Vector Laboratories, Burlingame, California, USA) and kept in the dark until visualization of slides with a confocal microscope.
Quantification of BrdU and TH labelled cells
The BrdU- and TH-positive cells were visualized using a fluorescent microscope (Zeiss Axiophot Microscope, Carl Zeiss Canada Ltd., Toronto, Ontario, Canada) at 20× magnification with the aid of an Olympus DP70 (Olympus, Center Valley, Pennsylvania, USA) camera. Positive cells were counted in the 4 and 7 dpi goldfish brains to quantify cellular proliferation using ImageJ1.43I software (Rasband 1997–2014). The total number of TH- and BrdU-positive cells in goldfish brain sections were counted using unbiased, single-blind, counting of cells in comparable sections using the disector technique (Guillery 2002). For the TH and BrdU counting, five sections of the ventral telencephalic area at 4 and 7 dpi were analyzed per fish (n = 5 per group) where cells were counted the entire length of the ventricular surface along the ventral-dorsal axis of the left brain hemisphere using a specific counting frame of a width of ∼ 680 ± 80 μm stretching laterally from the ventricular surface to the apical surface. Labelled cells were localized according to the goldfish brain atlas of Peter and Gill (1975) and Hornby et al. (1987). Cell counting was confined within neuroanatomical borders of the target area. The total number of positive cells between the treatment group and control group were first tested for normality and then statistically compared using a Student’s t test (two-tailed) in SPSS Statistics (version 21). p-values ≤0.05 were considered statistically significant. All data are presented as mean + SEM.
Results
Effects of MPTP on female goldfish swimming behaviour
Female goldfish were treated with MPTP and their swimming movements were measured following MPTP injection. As shown in Fig. 1, control goldfish traveled an average of ∼1250 cm/5 min and rested for ∼14 s during the test period. However, there was an observable decrease in total distance traveled and an increase in resting time following the injection of MPTP. At 3 dpi of MPTP goldfish decreased distance traveled by ∼85% (p < 0.0001) (Fig. 1). There was a significant increase in resting time (p = 0.0011) at 3 dpi (Fig. 1b). Thereafter, the total distance traveled by MPTP-treated goldfish started to recover towards control levels at 7 dpi but was still significantly different compared with control (p = 0.0178). Reversible changes were evident for the resting time, which approached control levels of 7 dpi (p = 0.2981).
Fig. 1.
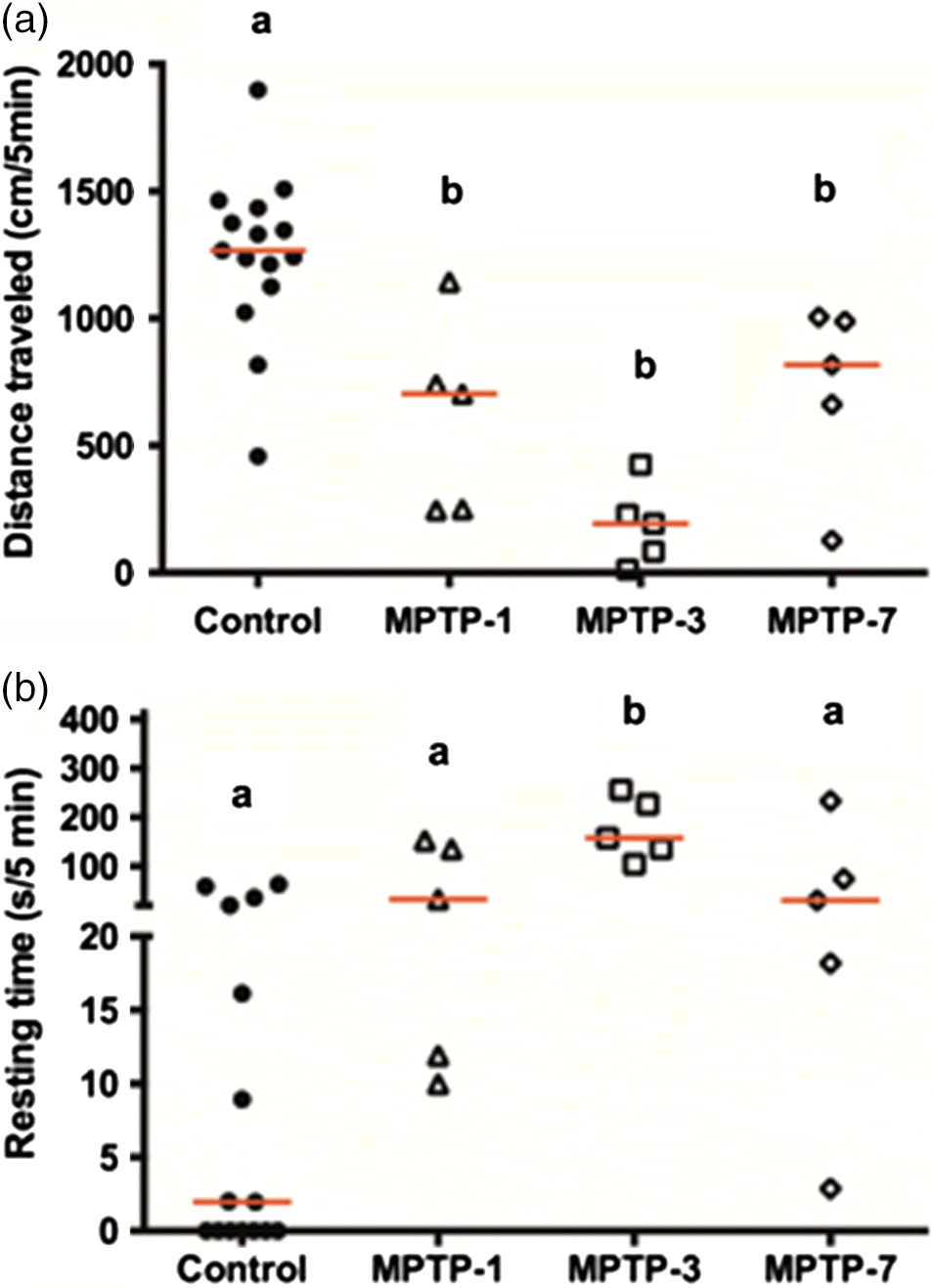
Effects of MPTP on TH and BrdU immunoreactivity in the female goldfish ventral telencephalon
Confocal imaging of control and MPTP-treated female goldfish brain sections at 4 and 7 dpi revealed TH- and BrdU-positive cell labelling in the Vd of the telencephalon. Figure 2b shows BrdU-positively labelled cells (red) located laterally and dorsally from the ventricular surface to the Vd area at 4 dpi of MPTP. Confocal imaging also revealed that BrdU-positive cells are colocalized with DAPI (blue nuclear stain) thereby indicating that BrdU was incorporated into newly generated cells in the female goldfish brain (Fig. 3a). Several double-labelled BrdU- and TH-positive cells were also found in the 4 dpi of MPTP fish group (Figs. 3b and 3c).
Fig. 2.
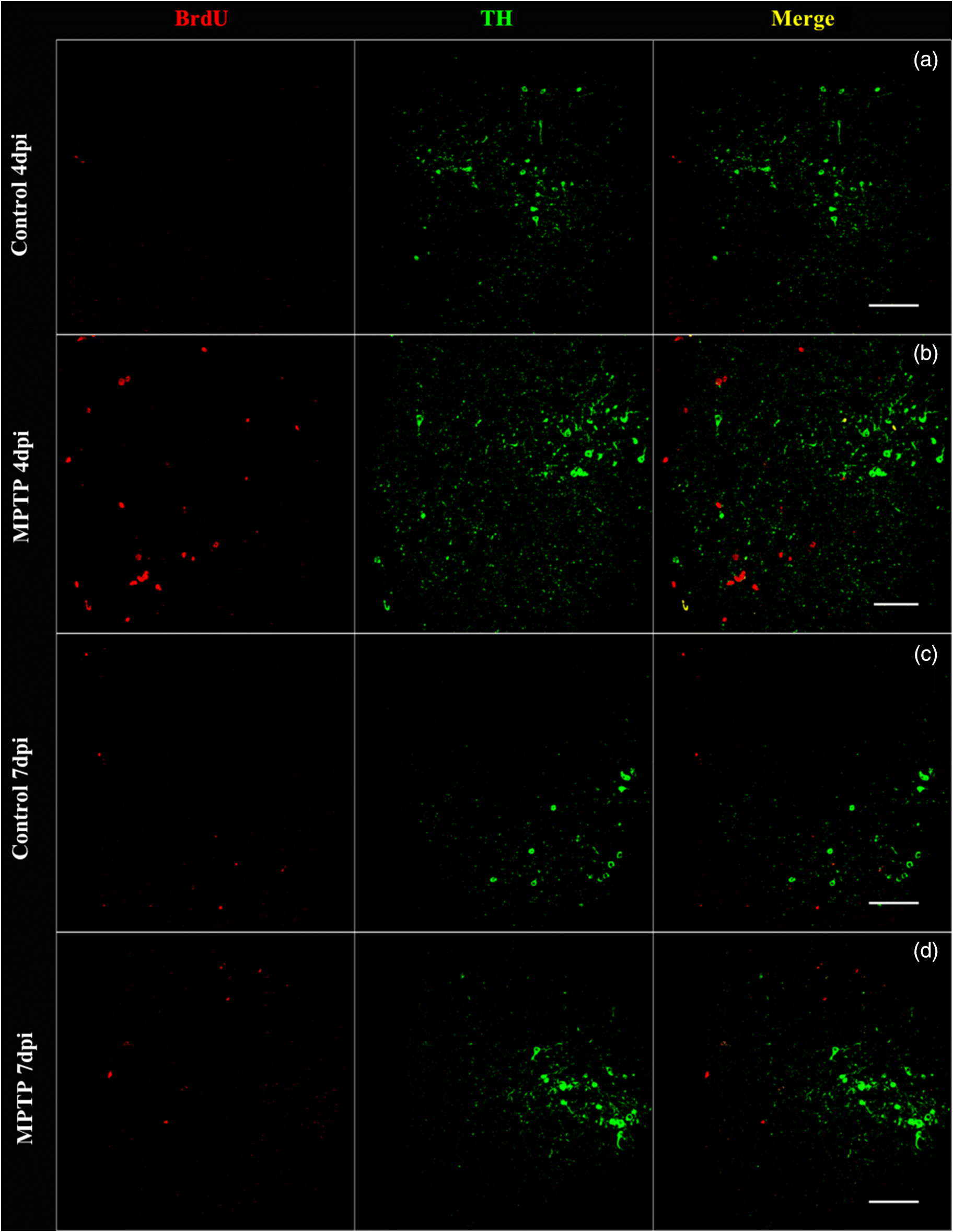
Fig. 3.
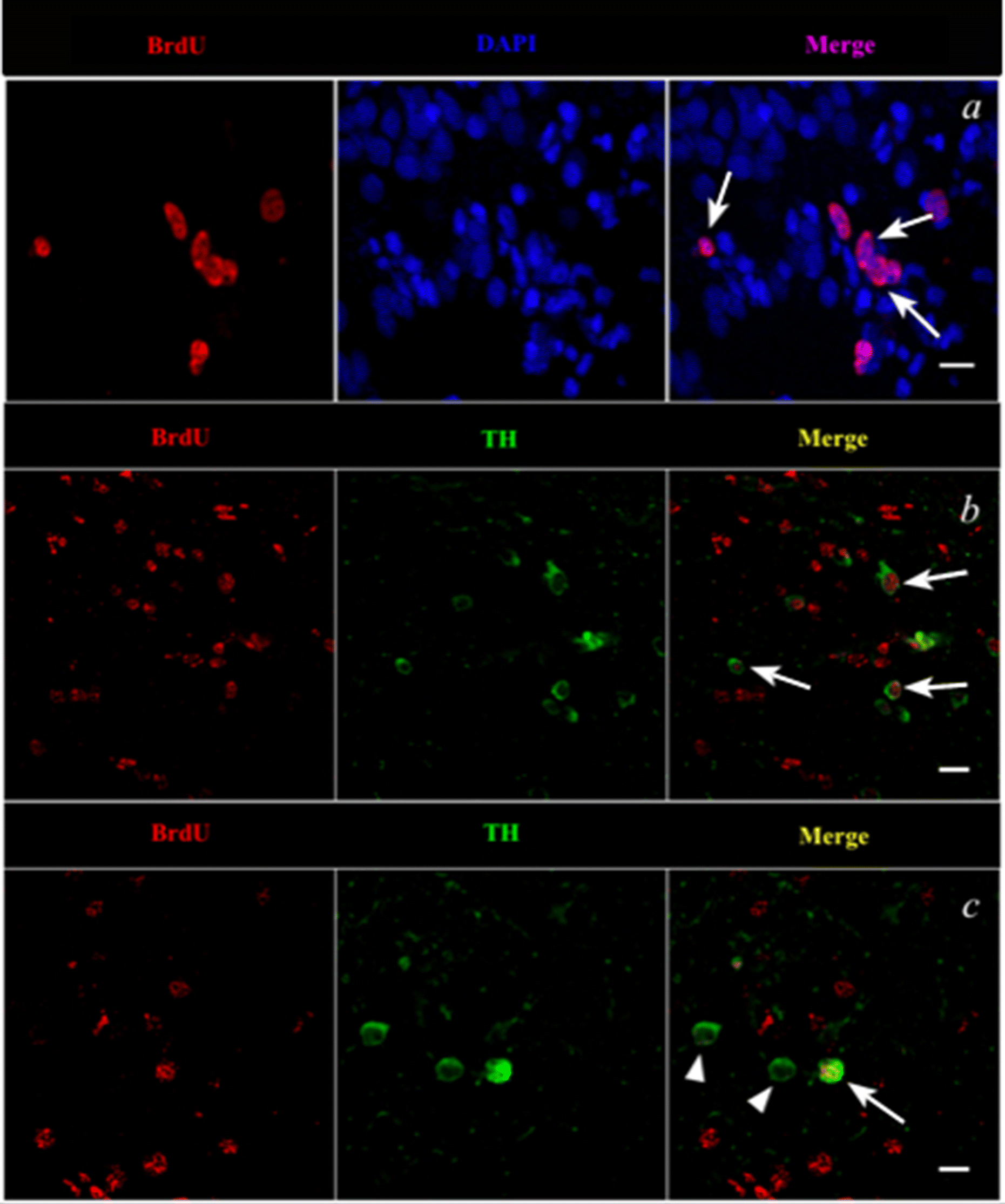
The total number of TH-positive neurons in the ventral telencephalon, which includes the Vd, was counted. At 4 dpi, TH-positive cells significantly decreased by 27% compared with control fish (p = 0.02) (Fig. 4a). No significant differences in TH-immunolabelling were observed at 7 dpi (p = 0.69) compared with controls (Fig. 4c). At 4 dpi, BrdU-positive cells significantly increased ∼1.9-fold compared with control fish (p = 0.02) (Fig. 4b). In contrast, no significant differences in BrdU immunolabelling were observed at 7 dpi (p = 0.69) (Fig. 4d).
Fig. 4.
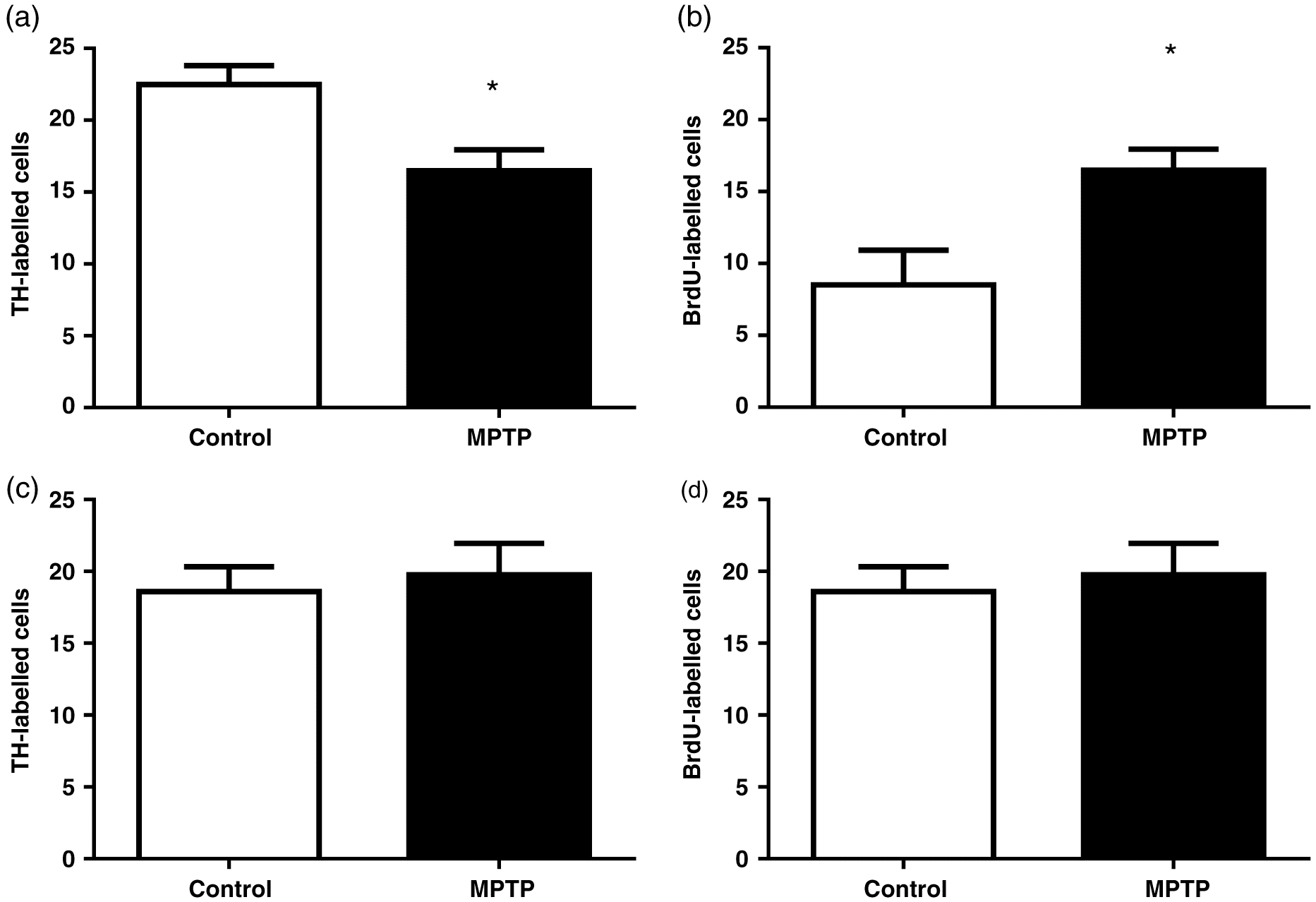
TH and GFAP immunoreactivity in the female goldfish ventral telencephalon
Immunoreactivity of TH and GFP in control goldfish ventral telencephalon, a region negatively impacted by MPTP toxicity (Goping et al. 1995) and one of which contains large amounts of RGCs (Pellegrini et al. 2016), reveals a close anatomical relationship between catecholamine neurons (TH) and GFAP fibers (∼5 μm) (Fig. 5). Dense GFAP immunoreactivity (red) was observed along the surface of the telencephalon ventricle with fibers extending ventrally towards the Vd where TH-immunoreactive catecolaminergic cells (green) are located.
Fig. 5.
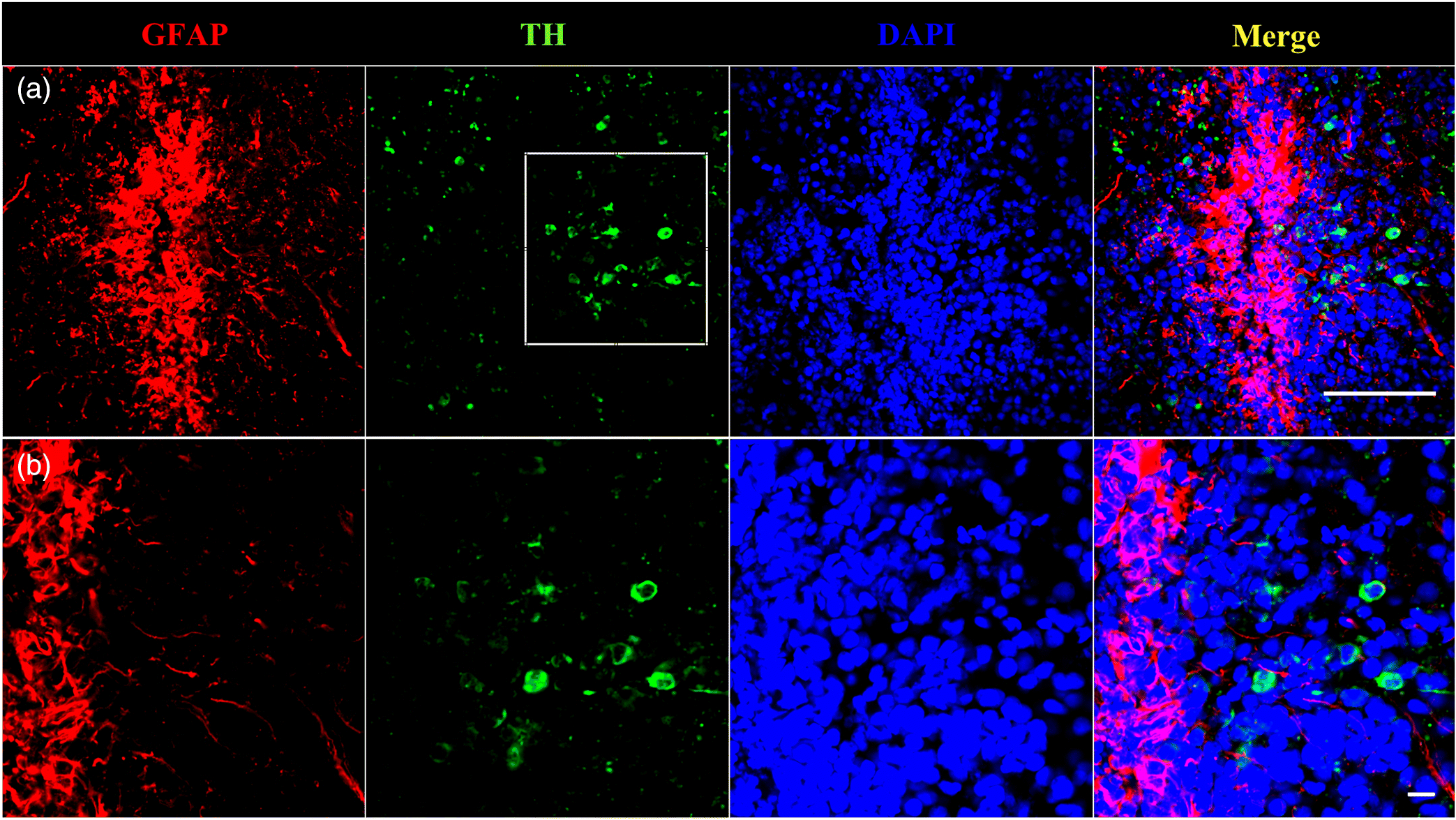
Discussion
The behavioural analysis indicated that goldfish treated with MPTP exhibit PD motor deficits consistent with previous reports (Pollard et al. 1992; Adeyemo and Youdim 1993; Weinreb and Youdim 2007). A significant ∼85% decrease in total distance traveled was observed in MPTP-treated goldfish 3 dpi, coincident with the maximal loss of DA in the forebrain, which has been reported previously (Poli et al. 1992; Pollard et al. 1992; Lu et al. 2014). Youdim et al. 1992 also reported profound bradykinesia in goldfish at 3 dpi of MPTP. We also observed a significant increase in total resting time in MPTP-injected goldfish at 3 dpi. Goldfish began significant recovery in total swimming distance and resting time by 7 dpi. A similar recovery of total distance traveled and resting time was reported at 7 and 5 dpi, respectively (Weinreb and Youdim 2007). The recovery of normal motricity suggests a recovery of neurotransmitter levels or neuronal function, i.e., DA, and thus the ability of goldfish to reestablish important biological behaviours following neurotoxic insult.
The TH-positive cells in the ventral telencephalon were depleted by MPTP at 4 dpi. The greatest decrease of forebrain DA content following MPTP injection is reached at 3–5 d (Pollard et al. 1992; Adeyemo et al. 1993; Sloley and McKenna 1993; Goping et al. 1995; Hibbert et al. 2004) coincident with TH depletion observed in the goldfish ventral telencephalon in this study. The TH immunoreactivity was quantified in the goldfish ventral telencephalon and was significantly decreased by 27% at 4 dpi of MPTP. Pollard et al. (1996) and Goping et al. (1995) also reported similar decreases in TH-immmunoreactivity at 4 d after MPTP treatment. Goldfish have also been reported to recover DA brain content to baseline levels at 7–8 dpi of MPTP (Pollard et al. 1992; Adeyemo et al. 1993; Popesku 2009). Remarkably, we observed a significant 1.9-fold increase in BrdU-labelled cells at 4 d following MPTP, but no significant differences were observed at 7 dpi in comparison with controls. This indicates that goldfish have a rapid recovery from MPTP toxicity starting at 4 dpi. Furthermore, BrdU-positive cell counts returned to control levels at 7 dpi, providing further evidence that neuroregeneration occurs rapidly after the neurotoxic insult in this species. Future studies are needed to determine if the rate of cellular proliferation returns to control levels following MPTP injection.
The short-term experiments conducted in this present study, where BrdU was injected 24 h prior to the 4 dpi of MPTP, allowed for sufficient time for BrdU to be available for incorporation into newly generated cells. Previous studies have shown that BrdU is readily available for uptake for ∼4 h after intraperitoneal injection (Zupanc and Horschke 1995). Furthermore, the length of the cell cycle in the adult teleost Astatotilapia burtoni (Günther, 1894) was estimated to be ∼25 h using proliferating cell nuclear antigen (PCNA) and BrdU labelling in the retina (Mack and Fernald 1997). Based on these studies, the positively labelled BrdU cells in the goldfish telencephalon likely label proliferative cells and their progeny if cellular division occurred. The longer-term experiment, where BrdU was available for a total of 4 d post-injection, proliferative cells that are actively dividing likely went through several cell cycles. Therefore the BrdU observed at this time point would likely label several cellular generations and also the original cell progeny. In this present study, we observed proliferation and lateral and dorsal migration of BrdU-positive cells towards the Vd area at 4 dpi. These newborn cells likely originated from the proliferative cellular pools along the ventricular surface where a large number of RGCs that can act as neural stem cells reside (Zupanc and Clint 2003; Mahler and Driever 2007; Pellegrini et al. 2007; März et al. 2010; Zupanc and Sîrbulescu 2011). At 7 dpi, the majority of proliferative cells remained near the ventricular area in the proliferative cell pools. A future experiment should be conducted to explore the possibility that BrdU remains in the goldfish brain for a longer period of time thus explaining why proliferative cells remained near the ventricle at 7 dpi.
Based on the observations of BrdU-positive cells in this study, we postulate that proliferative cells are completing or have completed their migration to the Vd following MPTP insult shortly after 4 dpi. Previous studies have reported the occurrence of rapid migration of newborn cells following injury based on BrdU cellular labelling. For example, cellular proliferation in the zebrafish cerebellum following injury was observed where cells migrated to their final destination of the granular cell layer after 10 d (Zupanc et al. 2005). Ampatzis and Dermon (2007) also found BrdU-labelled cells in the migration state at 24 h post-injury in the zebrafish cerebellum. Another study of spinal cord injury in A. leptorhynchus reported a several fold increase in the rate of cell proliferation starting at 1 d post-injury and lasting several weeks (Zupanc and Ott 1999; Reimer et al. 2008; Sîrbulescu et al. 2009). It is important to note that seasonality and environmental conditions can influence the rate of cellular proliferation in the brain as observed in the electric fish Brachyhypopomus gauderio Giora and Malabarba, 2009 where breeding fish had 3–7 times more BrdU-positive cells in the brain compared with nonbreeding fish (Dunlap et al. 2011). Seasonality could also impact the rate of motor recovery observed in the behavioural experiment as fish with different reproductive stages were used.
Remarkably, we observed some colocalization of BrdU- and TH-positive cells in the Vd at 4 dpi. This provides direct evidence that TH-positive neurons are regenerating in the region exhibiting neuronal depletion following MPTP injections. There were also BrdU-positive cells that did not stain for TH. These cells could still be migrating to their final target site and did not yet express TH. It is also possible that other non-TH expressing neuronal cell types could be differentiating in the Vd following a neurotoxic insult. Future studies are needed to fully characterize these newborn cells in the telencephalon.
The close proximity (<5 μm) of RGC fibers and TH-positive cells suggests that the newly generated cells may be migrating along RGC fibers to their final destination. Xing et al. 2017 showed that the injection of MPTP in goldfish induces an upregulation of mRNAs for glial cell-derived neurotrophic factor and brain-derived neurotrophic factor as well as genes involved in DA neuron development such as TH, dopamine transporter (slc6a3), and paired-like homeodomain transcription factor 3 (pitx3) in both the goldfish hypothalamus and telencephalon. These neurotrophic factors are produced by glial cells in teleosts and are probably involved in DA neuronal survival, decreasing DA neuronal apoptosis and promoting axonal growth and regeneration (Lin et al. 1993; Lykissas et al. 2007; Pellegrini et al. 2007; Yasuhara et al. 2007; Xing et al. 2017). This provides further evidence that the newly generated TH cells are most likely regenerating from a RGC pool along the ventricular surface of the telencephalon and migrating to damaged sites, e.g., the Vd, to restore catecholaminergic function (Fig. 6).
Fig. 6.
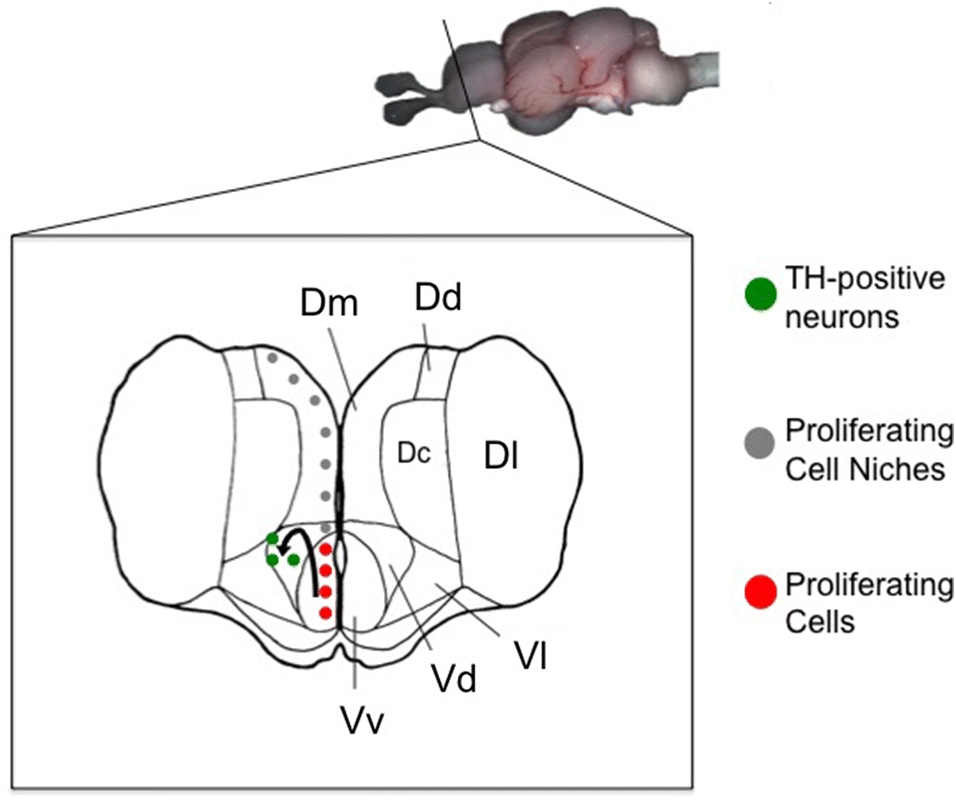
Acknowledgements
This research was supported by the NSERC Discovery program (VLT) and the University of Ottawa Research Chair in Neuroendocrinology (VLT). The authors greatly appreciate the help of Dr. Olivier Kah and Marie-Madeleine Gueguen from the Research Institute in Health, Environment and Occupation, Université de Rennes 1, France, for their technical guidance with experiments and microscopy expertise. We would like to thank Marilyn Vera Chang and Antony D. St-Jacques for providing assistance with the fish tracking software. We would also like to acknowledge the help of Bill Fletcher and Christine Archer for maintenance and care of the goldfish and Wei Seah for his help with experiments and fish husbandry.
References
Adeyemo OM, Youdim MBH, Markey SP, Markey CJ, and Pollard HB. 1993. l-Deprenyl confers specific protection against MPTP-induced Parkinson’s disease-like movement disorder in the goldfish. European Journal of Pharmacology, 240: 185–193.
Adolf B, Chapouton P, Lam CS, Topp S, Tannhäuser B, Strähle U, et al. 2006. Conserved and acquired features of adult neurogenesis in the zebrafish telencephalon. Developmental Biology, 295: 278–293.
Ampatzis K, and Dermon CR. 2007. Sex differences in adult cell proliferation within the zebrafish (Danio rerio) cerebellum. European Journal of Neuroscience, 25: 1030–1040.
Beattie MS, Farooqui AA, and Bresnahan JC. 2000. Review of current evidence for apoptosis after spinal cord injury. Journal of Neurotrauma, 17(10): 915–925.
Becker CG, and Becker T. 2007. Growth and pathfinding of regenerating axons in the optic projection of adult fish. Journal of Neuroscience Research, 85: 2793–2799.
Becker CG, and Becker T. 2008. Adult zebrafish as a model for successful central nervous system regeneration. Restorative Neurology and Neuroscience, 26: 71–80.
Bernstein JJ. 1964. Relation of spinal cord regeneration to age in adult goldfish. Experimental Neurology, 9: 161–174.
Burns RS, Chiueh CC, Markey SP, Ebert MH, Jacobowitz D, and Kopin IJ. 1983. A primate model of parkinsonism: selective destruction of dopaminergic neurons in the pars compacta of the substantia nigra by N-methyl-4-phenyl-l,2,3,6-tetrahydropyridine. Proceedings of the National Academy of Sciences of the U.S.A, 80: 4546–4550.
Chapouton P, Webb KJ, Stigloher C, Alunni A, Adolf B, Hesl B, et al. 2011. Expression of hairy/enhancer of split genes in neural progenitors and neurogenesis domains of the adult zebrafish brain. The Journal of Comparative Neurology, 519: 1748–1769.
Chiueh CC, Markey SP, Burns R, Johannensen JN, Jacobowitz D, and Kopin IJ. 1984. Neurochemical and behavioral effects of 1-methyl-4-phenyl-l,2,3,6-tetrahydropyridine (MPTP) in rat, guinea pig, and monkey. Psychopharmacology Bulletin, 20: 548–553.
Dunlap KD, Silva AC, and Chung M. 2011. Environmental complexity, seasonality and brain cell proliferation in a weakly electric fish, Brachyhypopomus gauderio. Journal of Experimental Biology, 214: 794–805.
Forlano PM, Deitcher DL, Myers DA, and Bass AH. 2001. Anatomical distribution and cellular basis for high levels of aromatase activity in the brain of teleost fish: aromatase enzyme and mRNA expression identify glia as source. The Journal of Neuroscience, 21: 8943–8955.
Ganz J, Kaslin J, Hochmann S, Freudenreich D, and Brand M. 2010. Heterogeneity and Fgf dependence of adult neural progenitors in the zebrafish telencephalon. Glia, 58: 1345–1363.
Goping G, Pollard HB, Adeyemo OM, and Kuijpers GAJ. 1995. Effect of MPTP on dopaminergic neurons in the goldfish brain: a light and electron microscope study. Brain Research, 687: 35–52.
Guillery RW. 2002. On counting and counting errors. The Journal of Comparative Neurology, 447: 1–7.
Hallman H, Lange J, Olson L, Strömberg I, and Jonsson G. 1985. Neurochemical and histochemical characterization of neurotoxic effects of 1-methyl-4-phenyl-1,2,3,6-tetrahydropyridine on brain catecholamine neurones in the mouse. Journal of Neurochemistry, 44: 117–127.
Hibbert B, Fung I, McAuley R, Larivière K, MacNeil B, Bafi-Yeboa N, et al. 2004. Increased GAD67 mRNA levels are correlated with in vivo GABA synthesis in the MPTP-treated catecholamine-depleted goldfish brain. Molecular Brain Research, 128: 121–130.
Hinsch K, and Zupanc GKH. 2007. Generation and long-term persistence of new neurons in the adult zebrafish brain: a quantitative analysis. Neuroscience, 146: 679–696.
Hornby PJ, Piekut DT, and Demski LS. 1987. Localization of immunoreactive tyrosine hydroxylase in the goldfish brain. The Journal of Comparative Neurology, 261: 1–14.
Kaslin J, Ganz J, Geffarth M, Grandel H, Hans S, and Brand M. 2009. Stem cells in the adult zebrafish cerebellum: initiation and maintenance of a novel stem cell niche. The Journal of Neuroscience, 29: 6142–6153.
Lam CS, Korzh V, and Strahle U. 2005. Zebrafish embryos are susceptible to the dopaminergic neurotoxin MPTP. European Journal of Neuroscience, 21: 1758–1762.
Levine RL. 1983. Widespread regeneration of central axons through the central nervous system of the goldfish. Developmental Brain Research, 9: 416–419.
Lin LF, Doherty DH, Lile JD, Bektesh S, and Collins F. 1993. GDNF: a glial cell line-derived neurotrophic factor for midbrain dopaminergic neurons. Science, 260: 1130–1132.
Liou AKF, Clark RS, Henshall DC, Yin X-M, and Chen J. 2003. To die or not to die for neurons in ischemia, traumatic brain injury and epilepsy: a review on the stress-activated signaling pathways and apoptotic pathways. Progress in Neurobiology, 69: 103–142.
Lu Z, Wang J, Li M, Liu Q, Wei D, Yang M, et al. 2014. 1H NMR-based metabolomics study on a goldfish model of Parkinson’s disease induced by 1-methyl-4-phenyl-1,2,3,6-tetrahydropyridine (MPTP). Chemico-Biological Interactions, 223: 18–26.
Lucchi R, Notari S, Pierantozzi S, Barnabei O, Villani L, and Poli A. 1998. Effect of 1-methyl-4-phenyl-1,2,3,6-tetrahydropyridine in goldfish cerebellum: neurochemical and immunocytochemical analysis. Brain Research, 782: 105–112.
Lykissas MG, Batistatou AK, Charalabopoulos KA, and Beris AE. 2007. The role of neurotrophins in axonal growth, guidance, and regeneration. Current Neurovascular Research, 4:143–151.
Mack AF, and Fernald RD. 1997. Cell movement and cell cycle dynamics in the retina of the adult teleost Haplochromis burtoni. The Journal of Comparative Neurology, 388: 435–443.
Mahler J, and Driever W. 2007. Expression of the zebrafish intermediate neurofilament nestin in the developing nervous system and in neural proliferation zones at postembryonic stages. BMC Developmental Biology, 7: 89.
März M, Chapouton P, Diotel N, Vaillant C, Hesl B, Takamiya M, et al. 2010. Heterogeneity in progenitor cell subtypes in the ventricular zone of the zebrafish adult telencephalon. Glia, 58: 870–888.
McKinley ET, Baranowski TC, Blavo DO, Cato C, Doan TN, and Rubinstein AL. 2005. Neuroprotection of MPTP-induced toxicity in zebrafish dopaminergic neurons. Molecular Brain Research, 141: 128–137.
Pellegrini E, Mouriec K, Anglade I, Menuet A, Le Page Y, Gueguen M-M, et al. 2007. Identification of aromatase-positive radial glial cells as progenitor cells in the ventricular layer of the forebrain in zebrafish. The Journal of Comparative Neurology, 501: 150–167.
Pellegirini E, Diotel N, Vaillant-Capitaine C, Maria RP, Gueguen M-M, Nasri A, et al. 2016. Steroid modulation of neurogenesis: focus on radial glial cells in zebrafish. The Journal of Steroid Biochemistry and Molecular Biology, 160: 27–36.
Peter RE, and Gill VE. 1975. A stereotaxic atlas and technique for forebrain nuclei of the goldfish, Carassius auratus. The Journal of Comparative Neurology, 159: 69–101.
Poli A, Guarnieri O, Facchinetti F, and Villani L. 1990. Effect of 1-methyl-4-phenyl-1,2,3,6-tetrahydropyridine (MPTP) in goldfish brain. Brain Research, 534: 45–50.
Poli A, Gandolfi O, Lucchi R, and Barnabei O. 1992. Spontaneous recovery of MPTP-damaged catecholamine systems in goldfish brain areas. Brain Research, 585: 128–134.
Pollard HB, Dhariwal K, Adeyemo OM, Markey CJ, Caohuy H, Levine M, et al. 1992. A parkinsonian syndrome induced in the goldfish by the neurotoxin MPTP. The FASEB Journal, 6: 3108–3116.
Pollard HB, Kuijpers GA, Adeyemo OM, Youdim MBH, and Goping G. 1996. The MPTP-induced parkinsonian syndrome in the goldfish is associated with major cell destruction in the forebrain and subtle changes in the optic tectum. Experimental Neurology, 142: 170–178.
Popesku JT. 2009. Dopaminergic regulation of gene expression in the neuroendocrine brain of the goldfish (Carassius auratus). Ph.D. thesis, University of Ottawa, Ottawa, Ontario. 208 p.
Rasband WS. 1997–2014. Image J. US National Institutes of Health, Bethesda, Maryland, [online]: Available from imagej.nih.gov/ij/.
Reimer MM, Sörensen I, Kuscha V, Frank RE, Liu C, Becker CG, et al. 2008. Motor neuron regeneration in adult zebrafish. The Journal of Neuroscience, 28: 8510–8516.
Rothenaigner I, Krecsmarik M, Hayes JA, Bahn B, Lepier A, Fortin G, et al. 2011. Clonal analysis by distinct viral vectors identifies bona fide neural stem cells in the adult zebrafish telencephalon and characterizes their division properties and fate. Development, 138: 1459–1469.
Sallinen V, Torkko V, Sundvik M, Reenilä I, Khrustalyov D, Kaslin J, et al. 2009. MPTP and MPP+ target specific aminergic cell populations in larval zebrafish. Journal of Neurochemistry, 108: 719–731.
Sîrbulescu RF, Ilies I, and Zupanc GKH. 2009. Structural and functional regeneration after spinal cord injury in the weakly electric teleost fish, Apteronotus leptorhynchus. Journal of Comparative Physiology A, 195: 699–714.
Sloley BD, and McKenna KF. 1993. 1-methyl-4-phenyl-1,2,3,6-tetrahydropyridine (MPTP), and γ-vinyl-γ-aminobutyric acid (γ-vinyl GABA) alter neurotransmitter concentrations in the nervous tissue of the goldfish (Carassius auratus) but not the cockroach (Periplaneta Americana). Neurochemistry International, 22: 197–203.
Stevenson JA, and Yoon MG. 1978. Regeneration of optic nerve fibers enhances cell proliferation in the goldfish optic tectum. Brain Research, 153: 345–351.
Stroh T, and Zupanc GKH. 1996. The postembryonic development of somatostatin immunoreactivity in the central posterior/prepacemaker nucleus of weakly electric fish, Apteronotus leptorhynchus: a double-labelling study. Developmental Brain Research, 93: 76–87.
Stuermer CAO. 1986. Pathways of regenerated retinotectal axons in goldfish. I. Optic nerve, tract and tectal fascicle layer. Journal of Embryology and Experimental Morphology, 93: 1–28.
Stuermer CAO, Bastmeyer M, Bähr M, Strobel G, and Paschke K. 1992. Trying to understand axonal regeneration in the CNS of fish. Developmental Neurobiology, 23: 537–550.
Sullivan SA, Barthel LK, Largent BL, and Raymond PA. 1997. A goldfish Notch-3 homologue is expressed in neurogenic regions of embryonic, adult, and regenerating brain and retina. Developmental Genetics, 20: 208–223.
Vajda FJE. 2002. Neuroprotection and neurodegenerative disease. Journal of Clinical Neuroscience, 9: 4–8.
Weinreb O, and Youdim MBH. 2007. A model of MPTP-induced Parkinson’s disease in the goldfish. Nature Protocols, 2: 3016–3021.
Wullimann MF, and Mueller T. 2004. Teleostean and mammalian forebrains contrasted: evidence from genes to behavior. The Journal of Comparative Neurology, 475: 143–162.
Xing L, Goswami M, and Trudeau VL. 2014. Radial glial cell: critical functions and new perspective as a steroid synthetic cell. General and Comparative Endocrinology, 203: 181–185.
Xing L, McDonald H, Da Fonte DF, Gutierrez-Villagomez JM, and Trudeau VL. 2015. Dopamine D1 receptor activation regulates the expression of the estrogen synthesis gene aromatase B in radial glial cells. Frontiers in Neuroscience, 9: 310
Xing L, Venables MJ, and Trudeau VL. 2017. Role of aromatase and radial glial cells in neurotoxin-induced dopamine neuron degeneration and regeneration. General and Comparative Endocrinology, 241: 69–79.
Yamamoto K, Ruuskanen JO, Wullimann MF, and Vernier P. 2011. Differential expression of dopaminergic cell markers in the adult zebrafish forebrain. The Journal of Comparative Neurology, 519: 576–598.
Yasuhara T, Shingo T, and Date I. 2007. Glial cell line-derived neurotrophic factor (GDNF) therapy for Parkinson’s disease. Acta Medica Okayama, 61: 51–56.
Youdim MBH, Dhariwal K, Levine M, Markey CJ, Markey S, Caohuy H, et al. 1992. MPTP-induced “parkinsonism” in the goldfish. Neurochemistry International, 20: 275–278.
Zikopoulos B, Kentouri M, and Dermon CR. 2000. Proliferation zones in the adult brain of a sequential hermaphrodite teleost species (Sparus aurata). Brain, Behavior and Evolution, 56: 310–322.
Zupanc GKH, and Horschke I. 1995. Proliferation zones in the brain of adult gymnotiform fish: a quantitative mapping study. The Journal of Comparative Neurology, 353: 213–233.
Zupanc GKH, and Ott R. 1999. Cell proliferation after lesions in the cerebellum of adult teleost fish: time course, origin, and type of new cells produced. Experimental Neurology, 160: 78–87.
Zupanc GKH, and Clint SC. 2003. Potential role of radial glia in adult neurogenesis of teleost fish. Glia, 43: 77–86.
Zupanc GKH, and Sîrbulescu RF. 2011. Adult neurogenesis and neuronal regeneration in the central nervous system of teleost fish. European Journal of Neuroscience, 34: 917–929.
Zupanc GKH, Hinsch K, and Gage FH. 2005. Proliferation, migration, neuronal differentiation, and long-term survival of new cells in the adult zebrafish brain. The Journal of Comparative Neurology, 488: 290–319.
Information & Authors
Information
Published In
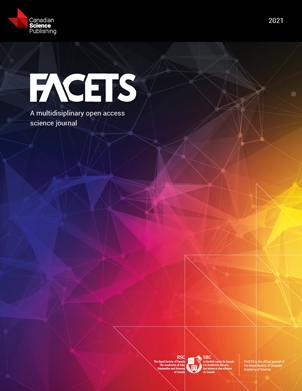
FACETS
Volume 3 • Number 1 • October 2018
Pages: 358 - 374
Editor: Imogen Coe
History
Received: 8 November 2017
Accepted: 21 January 2018
Version of record online: 4 April 2018
Copyright
© 2018 Venables et al. This work is licensed under a Creative Commons Attribution 4.0 International License (CC BY 4.0), which permits unrestricted use, distribution, and reproduction in any medium, provided the original author(s) and source are credited.
Data Availability Statement
All relevant data are within the paper.
Key Words
Sections
Subjects
Authors
Author Contributions
MJV and VLT conceived and designed the study.
MJV, LX, and CCE performed the experiments/collected the data.
MJV, LX, and CCE analyzed and interpreted the data.
VLT contributed resources.
MJV and VLT drafted or revised the manuscript.
Competing Interests
VLT is currently serving as a Subject Editor for FACETS, but was not involved in review or editorial decisions regarding this manuscript.
Metrics & Citations
Metrics
Other Metrics
Citations
Cite As
Maddie J. Venables, Lei Xing, Connor C. Edington, and Vance L. Trudeau. 2018. Neuronal regeneration in the goldfish telencephalon following 1-methyl-4-phenyl-1,2,3,6-tetrahydropyridine (MPTP) insult. FACETS.
3(1): 358-374. https://doi.org/10.1139/facets-2017-0119
Export Citations
If you have the appropriate software installed, you can download article citation data to the citation manager of your choice. Simply select your manager software from the list below and click Download.
Cited by
1. MPTP induces neurodegeneration by modulating dopaminergic activity in catfish brain
2. Quiescent Neural Stem Cells for Brain Repair and Regeneration: Lessons from Model Systems
3. A Comparative Perspective on Brain Regeneration in Amphibians and Teleost Fish