Trapped river otters (Lontra canadensis) from central Saskatchewan differ in total and organic mercury concentrations by sex and geographic location
Abstract
Introduction
Methods
Study area
Sample collection and processing
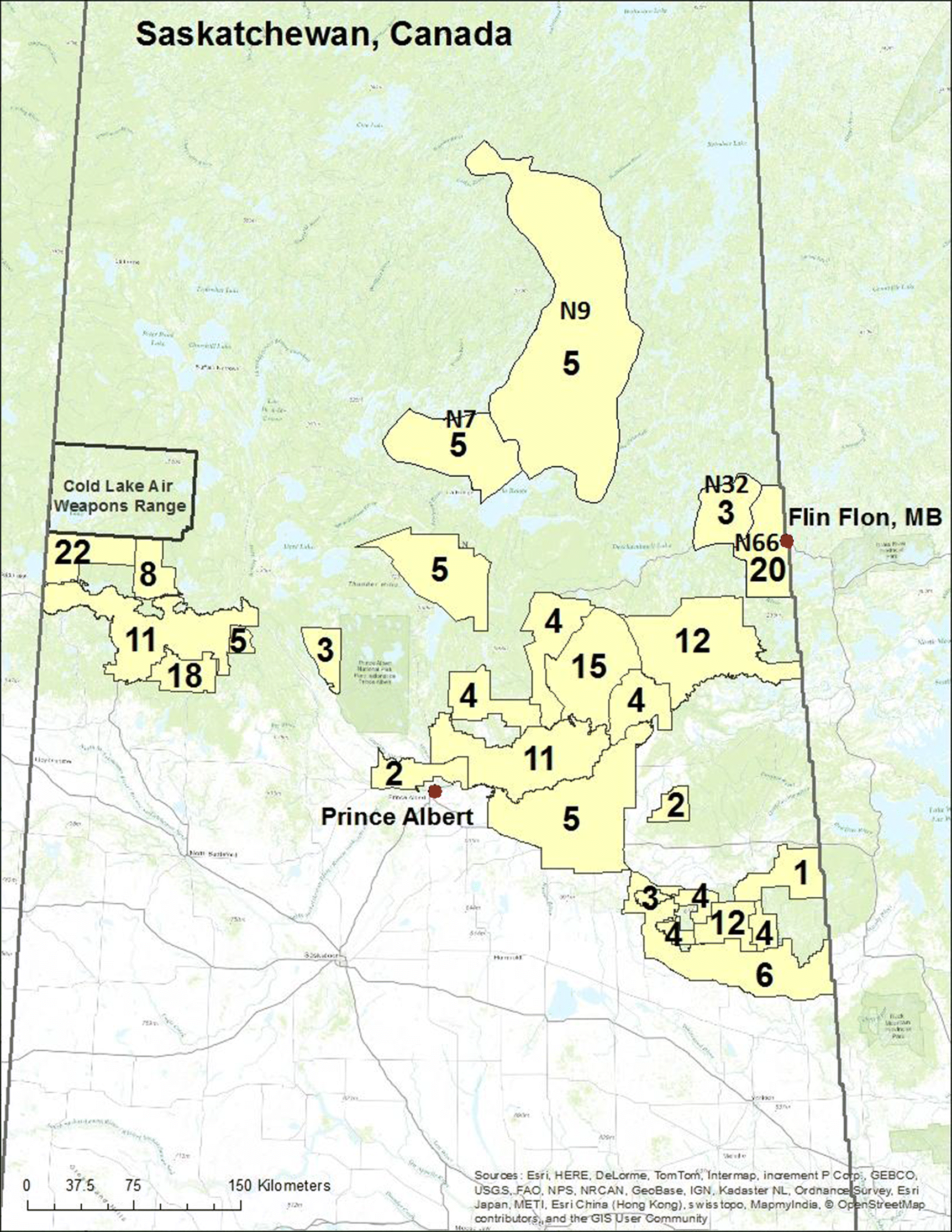
Variation of Hg in pelts
Organ subsampling
Tissue homogenization
THg and OHg analysis
Age determination
Data analysis
Results
Age and sex differences
Fur
THg
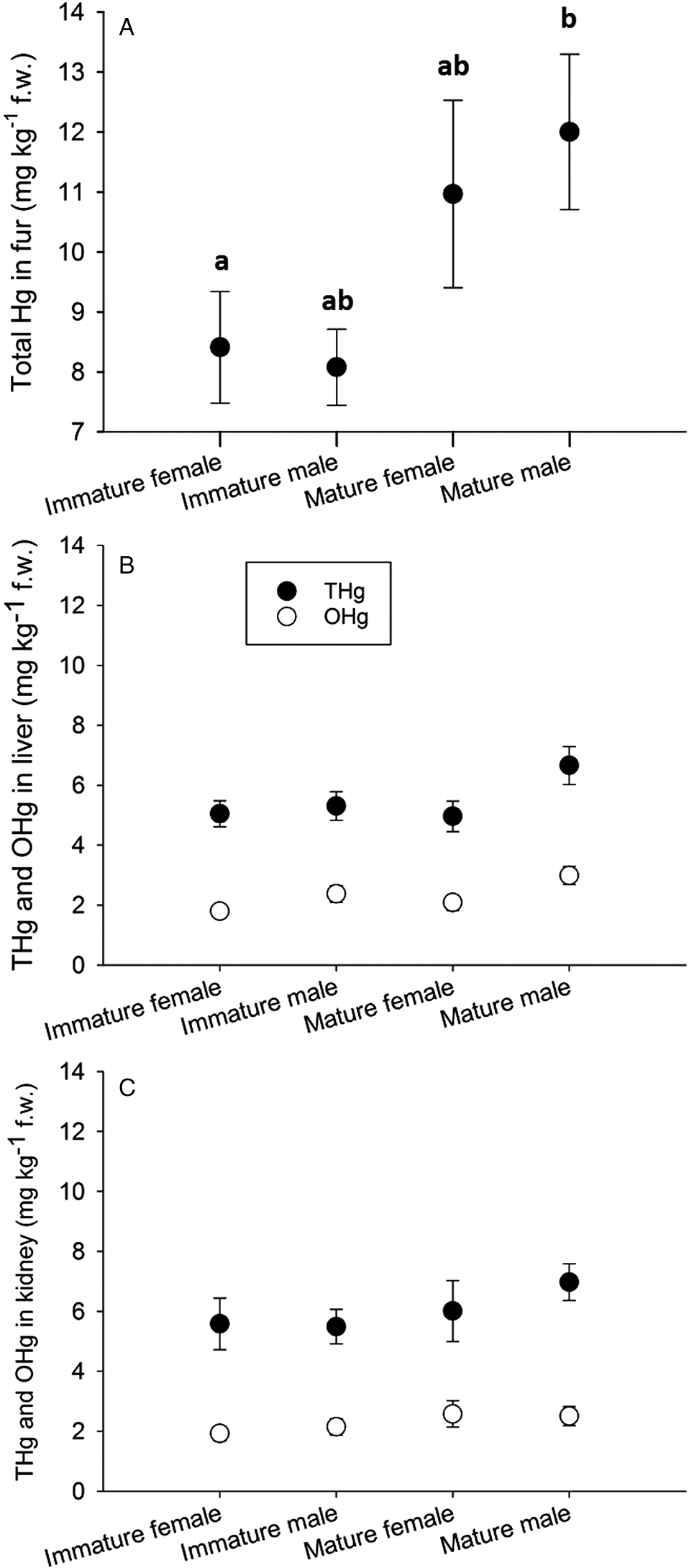
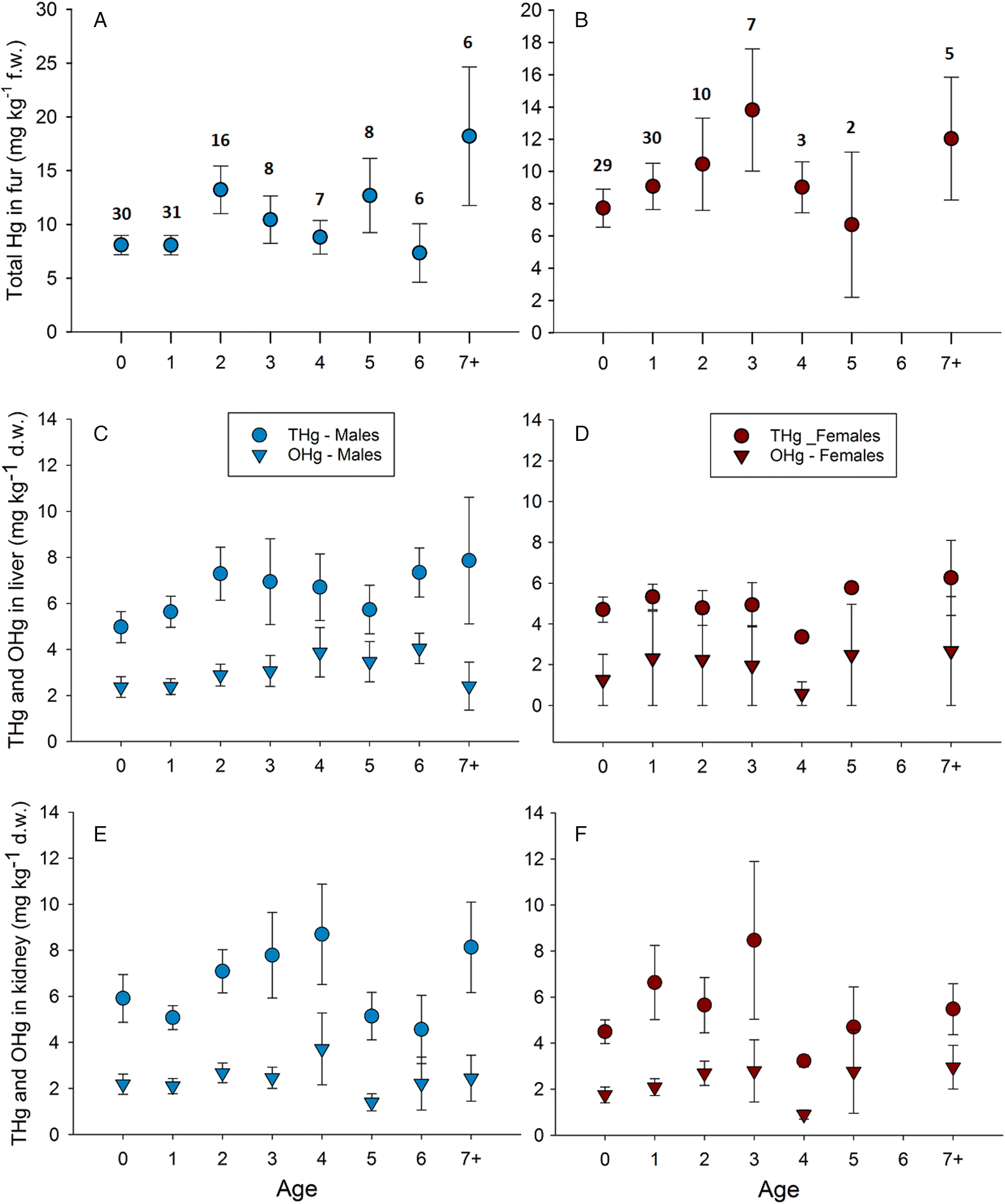
Relationship tested | Significant | Test results | Figure | |
---|---|---|---|---|
THg concentrations in fur | Age (males) | No | F7,102 = 1.46, p = 0.189 | Fig. 3A |
Age (females) | No | F6,77 = 0.93, p = 0.482 | Fig. 3B | |
Sex | No | F1,190 = 0.30, p = 0.584 | — | |
Maturity class | Yes | F1,190 = 9.84, p = 0.002 | Fig. 2A | |
Interaction effect of maturity and sex | No | F1,190 = 0.03, p = 0.874 | — | |
THg concentrations in liver | Age (males) | No | F7,101 = 1.01, p = 0.431 | Fig. 3C |
Age (females) | No | F6,76 = 0.94, p = 0.473 | Fig. 3D | |
Sex | No | F1,188 = 0.65, p = 0.423 | — | |
Maturity class | Yes | F1,188 = 4.09, p = 0.044 | Fig. 2B | |
Interaction effect of maturity and sex | No | F1,188 = 0.15, p = 0.700 | — | |
OHg concentrations in liver | Age (males) | No | F7,103 = 0.83, p = 0.567 | Fig. 3C |
Age (females) | Yes | F8,75 = 2.47, p = 0.031 | Fig. 3D | |
Sex | No | F1,191 = 3.26, p = 0.072 | — | |
Maturity class | No | F1,191 = 3.26, p = 0.072 | Fig. 2B | |
Interaction effect of maturity and sex | No | F1,191 = 0.15, p = 0.695 | — | |
%OHg (males) | No | rs = −0.02, n = 111, p = 0.841 | Fig. 4A | |
%OHg (females) | Yes | rs = −0.30, n = 84, p = 0.006 | Fig. 4B | |
THg concentrations in kidney | Age (males) | No | F7,103 = 1.51, p = 0.174 | Fig. 2E |
Age (females) | No | F6,77 = 0.55, p = 0.770 | Fig. 2F | |
Sex | No | F1,191 = 0.95, p = 0.332 | — | |
Maturity class | Yes | F1,191 = 4.50, p = 0.035 | Fig. 2C | |
Interaction effect of maturity and sex | No | F1,191 = 0.59, p = 0.443 | — | |
OHg concentrations in kidney | Age (males) | No | F7,104 = 0.60, p = 0.753 | Fig. 3E |
Age (females) | No | F6,77 = 0.87, p = 0.520 | Fig. 3F | |
Sex | No | F1,192 = 0.05, p = 0.818 | — | |
Maturity class | No | F1,192 = 3.15, p = 0.077 | Fig. 2C | |
Interaction effect of maturity and sex | No | F1,192 = 0.30, p = 0.582 | — | |
%OHg (males) | No | rs = −0.03, n = 113, p = 0.760 | — | |
%OHg (females) | No | rs = 0.01, n = 85, p = 0.900 | — |
Liver and kidney
THg
OHg
Variable | THg fur | THg liver | OHg liver | THg kidney | OHg kidney |
---|---|---|---|---|---|
THg fur | 1 | 0.734 | 0.395 | 0.685 | 0.439 |
THg liver | — | 1 | 0.510 | 0.816 | 0.472 |
OHg liver | — | — | 1 | 0.431 | 0.453 |
THg kidney | — | — | — | 1 | 0.575 |
OHg kidney | — | — | — | — | 1 |
Note: All p-values were <0.01; n = 192.
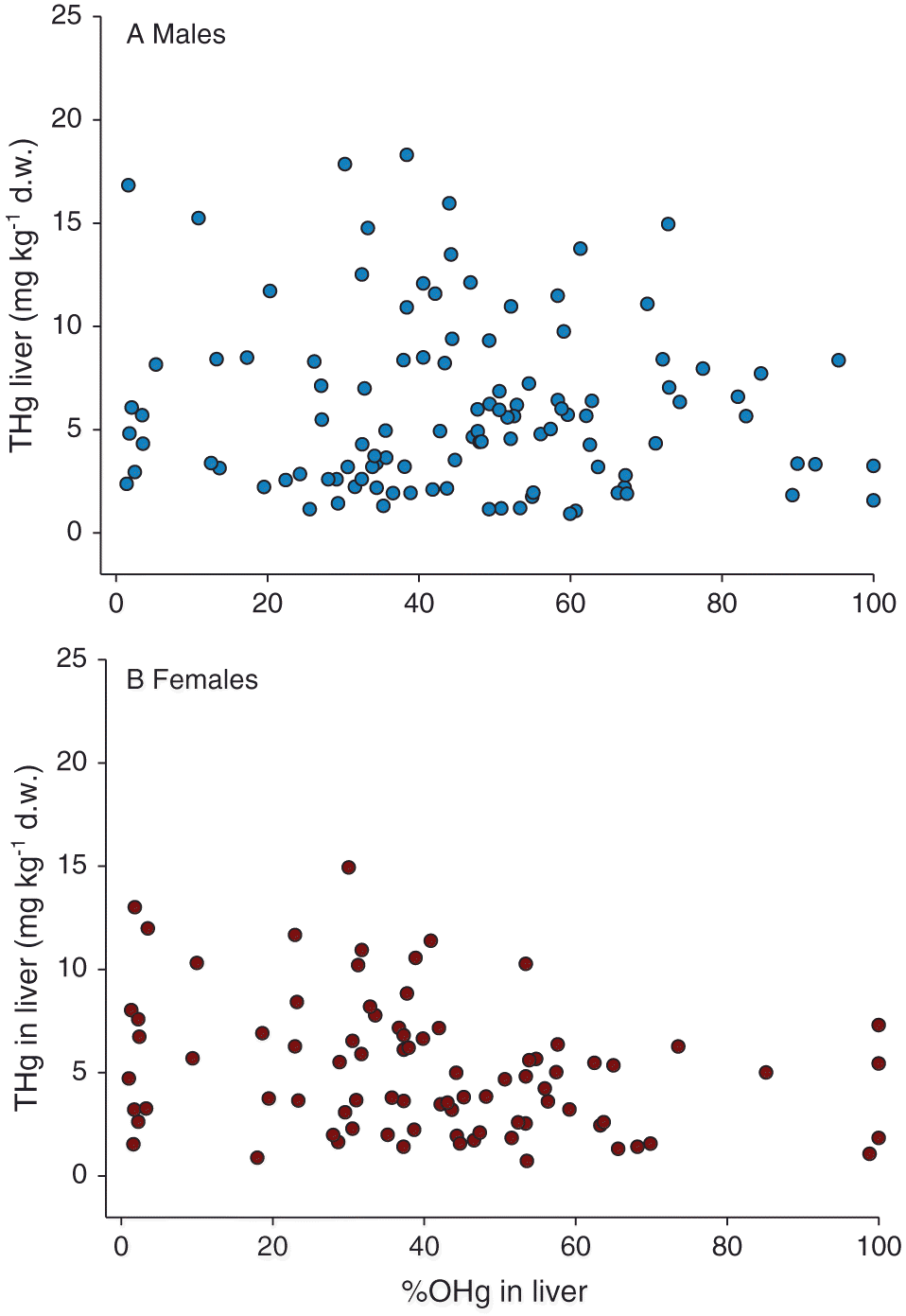
Geographic variability
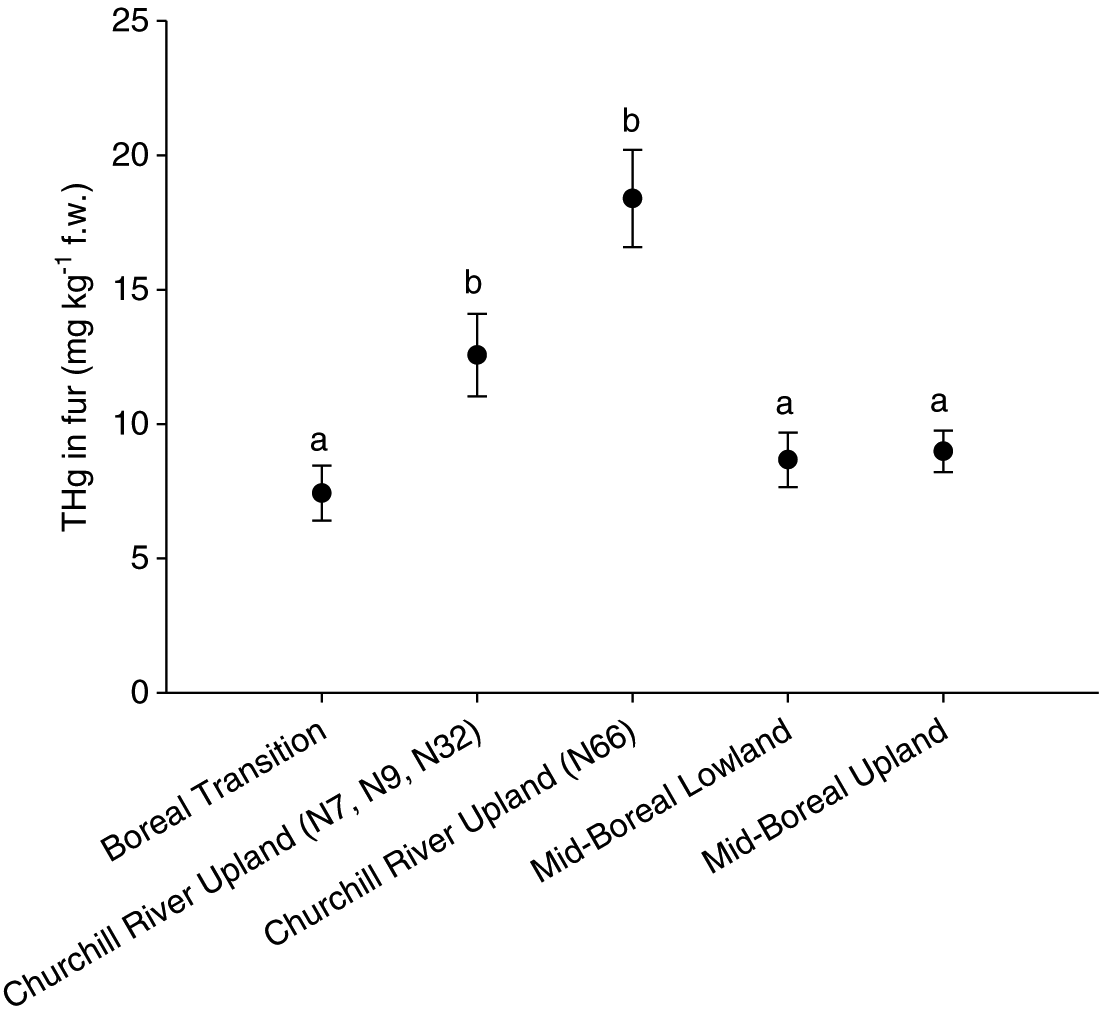
Discussion
Hg concentrations in tissues
Age and sex differences
Geographic variation in Hg levels
Conclusion
Acknowledgements
References
Supplementary material
- Download
- 350.35 KB
Information & Authors
Information
Published In
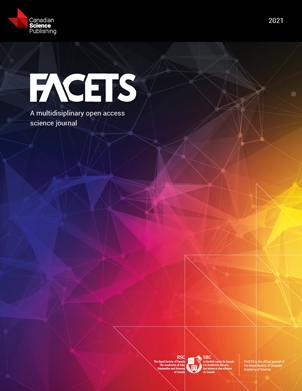
History
Copyright
Data Availability Statement
Key Words
Sections
Subjects
Authors
Author Contributions
Competing Interests
Metrics & Citations
Metrics
Other Metrics
Citations
Cite As
Export Citations
If you have the appropriate software installed, you can download article citation data to the citation manager of your choice. Simply select your manager software from the list below and click Download.