Canada's marine carbon sink: an early career perspective on the state of research and existing knowledge gaps
Abstract
Improving our understanding of how the ocean absorbs carbon dioxide is critical to climate change mitigation efforts. We, a group of early career ocean professionals working in Canada, summarize current research and identify steps forward to improve our understanding of the marine carbon sink in Canadian national and offshore waters. We have compiled an extensive collection of reported surface ocean air–sea carbon dioxide exchange values within each of Canada's three adjacent ocean basins. We review the current understanding of air–sea carbon fluxes and identify major challenges limiting our understanding in the Pacific, the Arctic, and the Atlantic Ocean. We focus on ways of reducing uncertainty to inform Canada's carbon stocktake, establish baselines for marine carbon dioxide removal projects, and support efforts to mitigate and adapt to ocean acidification. Future directions recommended by this group include investing in maturing and building capacity in the use of marine carbon sensors, improving ocean biogeochemical models fit-for-purpose in regional and ocean carbon dioxide removal applications, creating transparent and robust monitoring, verification, and reporting protocols for marine carbon dioxide removal, tailoring community-specific approaches to co-generate knowledge with First Nations, and advancing training opportunities for early career ocean professionals in marine carbon science and technology.
Introduction
Increases in greenhouse gas emissions due to human activity are driving adverse changes to human systems and ecosystems, including increases in biodiversity loss, food and water insecurity, and extreme weather events (IPCC 2023). To mitigate climate change-related risks, Canada must adhere to national and international greenhouse gas emission reduction strategies and environmental policies. Doing so requires careful accounting of Canada's carbon stocks and fluxes. To this end, we must improve our understanding of the ocean's role in the global carbon cycle. Understanding the variability of the marine carbon sink can better inform future scientific observational programs, climate forecasting, and net-zero emission pathways (Environment and Climate Change Canada 2020). Current estimates suggest that the global ocean has taken up approximately one quarter of the total anthropogenic (i.e., human-caused) carbon dioxide (CO2) emissions (Lindoso 2019; Friedlingstein et al. 2022). Yet, gaps in our knowledge of the spatial and temporal variability in the natural marine carbon sink limit our ability to assess potential future changes in this important process. Indeed, owing to a lack of continuous observations of surface ocean CO2 and air–sea CO2 fluxes, especially in high-latitude regions and during the winter season, the long-term variability of the physical and biological processes that contribute to the marine carbon sink remains poorly understood (McKinley et al. 2011; Fay and McKinley 2013; Wanninkhof et al. 2013). To address this knowledge gap, we must improve the spatial and temporal coverage of marine carbon flux observations (Aricò et al. 2021) and integrate new data with efforts to improve ocean biogeochemical modelling and climate projections. These tools should be used alongside other approaches from non-scientific viewpoints (e.g., traditional knowledge) to inform the co-development of climate change impact adaptation strategies and marine mitigation methods.
In Canada, current climate policy focuses on energy systems, infrastructure, transportation, and the terrestrial carbon sink. Presently, the marine carbon sink is excluded from climate policy considerations in the Pan-Canadian Framework on Clean Growth and Climate Change (Government of Canada 2016; Dion et al. 2021). However, the Canadian coastline is the largest in the world, touching three major ocean basins: the Pacific, Arctic, and Atlantic (Fig. 1). In these waters, both physics and biology cause the marine carbon sink to vary strongly over space and time (Laruelle et al. 2018; Fennel et al. 2019). As the data we have compiled will show, Canada's oceans are collectively considered a natural CO2 sink with large heterogeneity, making it difficult to incorporate the marine system into Canada's climate change mitigation plans, let alone the United Nations’ Framework Convention on Climate Change emissions accounting system (Dion et al. 2021). To measure the success of the Paris Agreement as part of the global stocktake (Peters et al. 2017), climate action and emission reduction targets must be adjusted to reflect variability in the marine carbon sink while considering the social equity of the resulting policies (Boyce 2018; Carley and Konisky 2020; Peng 2020).
Fig. 1.
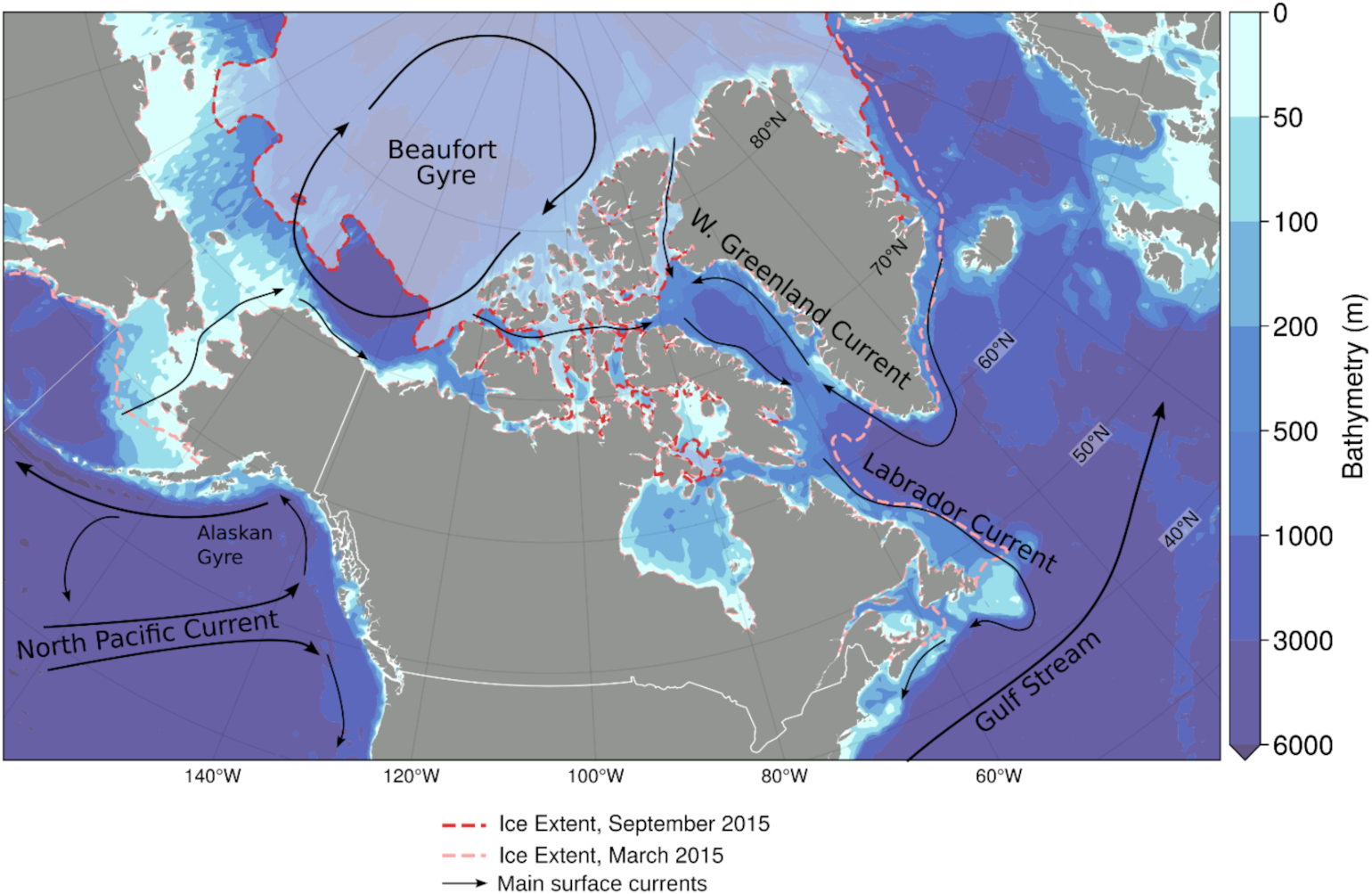
In reaching net-zero emissions, there is high demand from governments and businesses for carbon dioxide removal (CDR) projects, with many proposed in marine settings (Cooley et al. 2022). Proposed projects include artificially stimulating biological carbon drawdown or manipulating seawater properties to enhance CO2 absorption (GESAMP 2019; NASEM 2021). In western Canada, the Provincial Government of British Columbia has designated coastal blue carbon (i.e., carbon stored in marine systems) as a negative emissions “technology” aimed at meeting emission reduction goals (Government of British Columbia 2021). Other Canadian jurisdictions will likely follow suit (Drever et al. 2021; Fong and MacDougall 2023), with many start-up companies and carbon creditors rapidly moving into ocean CDR (Hurd et al. 2022). However, many proposed CDR approaches focus on CO2 removal from seawater (GESAMP 2019), instead of direct uptake from the atmosphere. While the resulting deficit in oceanic CO2 drives the transfer of CO2 from the atmosphere to the ocean, the timescale of re-equilibration varies from weeks to months and depends heavily on various environmental factors (e.g., gas transfer velocity, mixed layer depth, ratio between marine carbonate system chemical species, and water mass subduction; Wanninkhof et al. 2009; Jones et al. 2014). A firm understanding of processes driving carbon fluxes and establishing environmental baselines becomes critical to ensuring emerging ocean CDR techniques are robust, permanent, measurable, and verifiable. In the absence of such considerations, CDR approaches may simply involve moving CO2 between different oceanic carbon pools, which may help mitigate ocean acidification locally but does not lead to CO2 removal from the atmosphere, the latter being required for climate change mitigation.
As a consequence of the oceanic uptake of anthropogenic CO2, ocean acidification is an increasingly prominent threat to both marine ecosystems and shellfish aquaculture (Orr et al. 2005; Doney et al. 2012, 2020; IPCC 2013). For example, increased acidity negatively impacts marine organisms that build calcium carbonate shells or skeletons (Azetsu-Scott et al. 2010) (e.g., corals, bivalves, coccolithophores, and pteropods), which may have consequences for marine food webs (Fabry et al. 2008; Haigh et al. 2015), including the culturally and economically relevant species that rely on them. Key commercial species such as oysters, mussels, and lobsters are particularly vulnerable to ocean acidification effects (Barton et al. 2012; Ekstrom et al. 2015; McLean et al. 2018), jeopardizing Canadian aquaculture revenues of approximately $115 million per year (Fisheries and Oceans Canada 2019a) and fisheries revenues of $3.6 billion per year (Fisheries and Oceans Canada 2019b). Coastal communities, especially First Nations that have constitutionally protected rights to traditional harvests, will likely incur unquantifiable social, cultural, and economic losses through the consequences of ocean acidification. Some ocean CDR approaches offer associated ocean acidification mitigation co-benefits (e.g., ocean alkalinity enhancement; Bach et al. 2019). In Canada, the British Columbia Ocean Acidification and Hypoxia Action Plan will support commitments within the CleanBC Roadmap to 2030 to explore ocean CDR (Government of British Columbia 2022).
Coastal Indigenous communities, as rights and title holders, will disproportionately require ocean acidification mitigation strategies and be faced with evaluating ocean CDR project proposals (Lezaun 2021). Natural science research is not immune to or removed from the need for reconciliation to rebalance relationships with First Nations (Truth and Reconciliation Commission of Canada 2015), which can create a path forward based on trust and respect (McGregor 2018; Wong et al. 2020; Kovach 2021). Indigenous peoples have a deep understanding of the land and waterways that comprise their traditional territories and continue to require new information to adapt to climate change impacts. Collaborative efforts to bridge different knowledge systems (Indigenous and Western) can help solve complex climate adaptation and mitigation problems. However, there is no one-size-fits-all approach to integrating different knowledge systems (Rivers et al. 2023). These projects require meeting individual community needs in a tailored approach built on trust, and those needs vary between coasts and nations (Rivers et al. 2023).
The next generation of oceanographers will need to evolve ocean science research to aid in climate change mitigation and adaptation action while addressing truth and reconciliation with First Nations in Canada. Against the backdrop of unprecedented rates of change in the marine environment (Pörtner et al. 2019), these early career researchers are playing (and will continue to play) a critical role in creating and regulating monitoring, reporting, and verification (MRV) protocols for ocean CDR. Differentiating the immense background noise of natural variability (i.e., seasonal, interannual, and decadal), compounded with anthropogenic climate change impacts, to discern and monetize ocean CDR intervention requires complete marine carbon budgets (Legge et al. 2020). Following widespread public criticism over forestry-based carbon credits that did not lead to genuine atmospheric carbon reductions (Greenfield 2023), early career ocean scientists will face strong public scrutiny to ensure ocean CDR is real and durable.
In light of the challenges identified above, in this article, we provide an early career perspective on the state of research and necessary steps to improve our understanding of the marine carbon sink in Canadian national and offshore waters. First, we outline the current state of knowledge and major challenges to quantifying air–sea CO2 fluxes in each of Canada's three adjacent ocean basins (coastal and offshore), along with coast-specific Indigenous-led or co-led projects. In the Future Directions section, we present our recommendations for future research initiatives. We prescribe enhanced collaboration among the observational and modelling communities and strongly advocate for the co-generation of knowledge by scientists and First Nations. As an interdisciplinary cohort of graduate students and postdoctoral fellows spanning five major Canadian universities and seven different nationalities, this article offers firsthand insight into the perspectives and direction for the upcoming generation of Canadian carbon-flux research scientists and ocean professionals.
Canadian marine CO2 uptake
Here, we have compiled the most complete collection of reported air–sea CO2 flux data in Canadian and adjacent open-ocean waters (Fig. 2 and Table S1), drawn from 61 published studies (14 Pacific, 8 Atlantic, 29 Arctic, and 10 global). This compilation of data will act as a reference for future work and as a timestamp for monitoring efforts as future climate change impacts the variability and intensity of the marine carbon sink. Figure 2 summarizes air–sea CO2 flux density (i.e., the amount of CO2 moving between the atmosphere and surface ocean in a given area and time) estimates complied in Table S1 in Canadian waters from a range of observational studies, interpolation-based products, and biogeochemical models. Air–sea CO2 flux estimates based on marine carbon state variables other than the partial pressure of CO2 (i.e., dissolved inorganic carbon (DIC), alkalinity, and pH) have been excluded due to the elevated uncertainty of such calculations (Orr et al. 2018). In general, Canadian waters are a net sink for atmospheric CO2 (Fig. 2 and Table S1). However, given the current uncertainty attributed to each individual estimate, as well as the variability and time between estimates, we cannot yet quantify a “policymaker-relevant” value in terms of grams of CO2 uptake per year. The compiled estimates come from both inside Canada's exclusive economic zone (200 mile offshore limit) and beyond it, including the offshore open ocean regions adjacent to Canada's shelf seas. Table S1 also includes expanded Arctic coverage of air–sea CO2 flux estimates. The offshore regions were included based on the transboundary nature of ocean processes and their potential influence on fluxes along Canada's continental margins (Fig. 1), as well as Canada's proximity to monitoring for global stocktake efforts. This collection of air–sea CO2 flux estimates only addresses one component of building complete marine carbon budgets (Legge et al. 2020). Carbon fluxes between other stocks, including the water column (pelagic), seafloor sediments (benthic), and at the terrestrial-to-marine interface (river input), as well as fluxes of non-CO2 greenhouse gases (e.g., methane and nitrous oxide), are not the focus of this article.
Fig. 2.
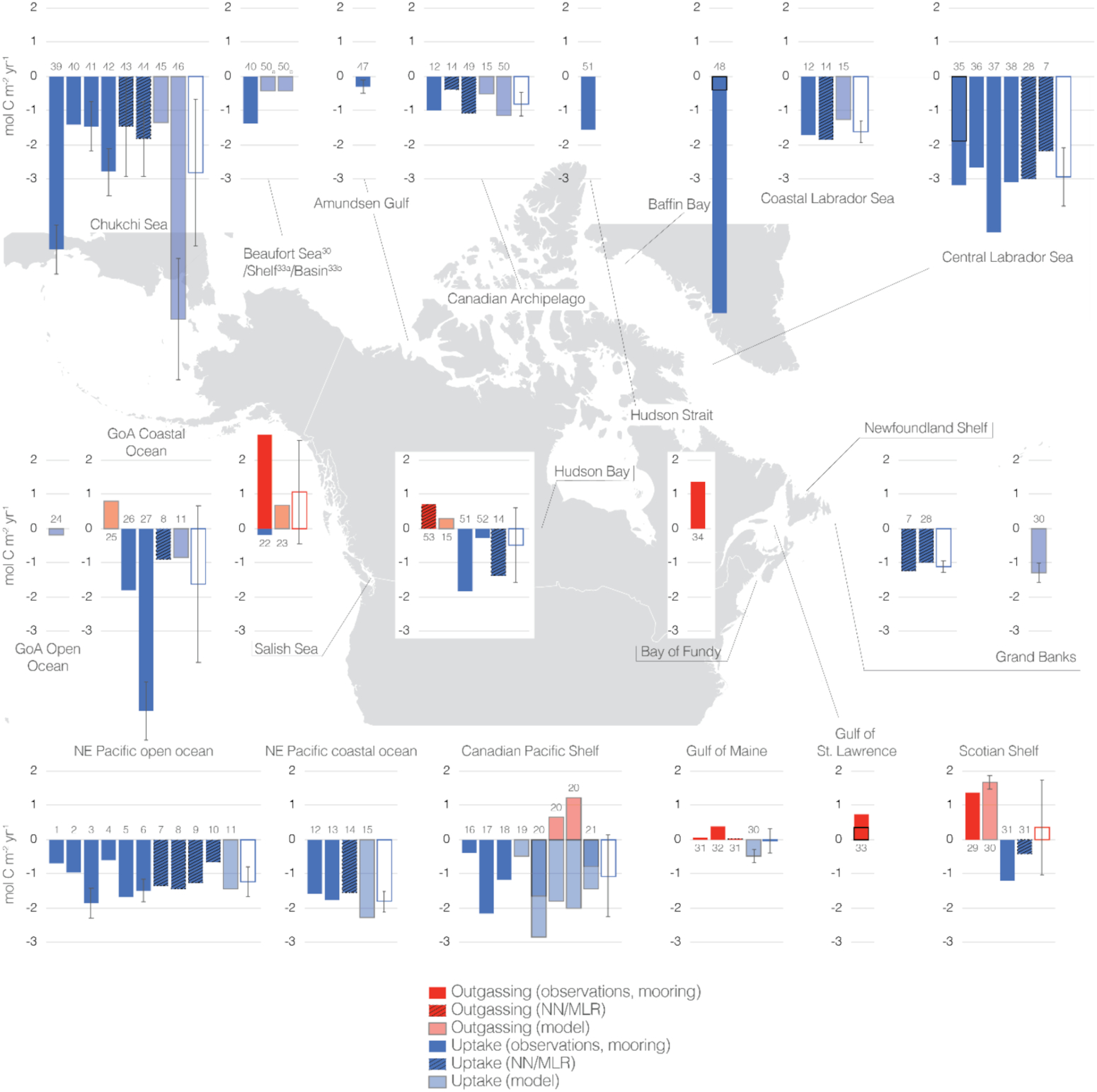
Canadian Pacific
The Subarctic Northeast Pacific appears to behave as a net sink for atmospheric CO2 at present (Fig. 2). However, the Canadian Pacific comprises diverse oceanographic regions—open ocean, continental shelf, marginal sea (i.e., Salish Sea), and numerous fjords—that contribute to large spatial variability in the magnitude and direction of the air–sea CO2 flux.
Published results suggest an overall open ocean CO2 sink of −1.1 ± 0.6 mol C m−2 year−1 (mean and standard deviation from Table S1). While persistently undersaturated with respect to atmospheric CO2, the seasonal amplitude in surface ocean CO2 is also relatively small (approximately 20 µatm; Sutton et al. 2017), mainly reflecting competing seasonal variability in sea surface temperature and DIC content (Wong et al. 2010; Sutton et al. 2017). Through the spring and summer, rising sea surface temperatures increase the partial pressure of surface ocean CO2 while biology consumes carbon in the iron-limited, high-nutrient low-chlorophyll region (Freeland et al. 1984; Dugdale and Wilkerson 1991; Martin et al. 1994; Aumont et al. 2003; Wong et al. 2010). Over the fall and winter, sea surface cooling decreases the partial pressure of surface ocean CO2 while mixed layer deepening mixes high-CO2 water into the surface (Wong et al. 2010). Observed long-term changes in CO2 fluxes show a clear increase in surface ocean CO2 generally consistent with, or slightly weaker than the atmospheric CO2 increase (Wong et al. 2010; Franco et al. 2021). Further north, the upwelling strength of the subpolar Alaskan Gyre has been shown to be the dominant control on surface carbonate chemistry seasonally (Fig. 1; Chierici et al. 2006; Palevsky et al. 2013; Brady et al. 2019) and on longer timescales (Hauri et al. 2021). Increased winter wind speeds drive stronger gyre upwelling, bringing CO2-rich subsurface waters to the surface leading to seasonal outgassing (Chierici et al. 2006). Over decadal timescales, this upwelling strength can dampen or accelerate apparent ocean acidification rates (Hauri et al. 2021).
Along the continental margin, strongly varying estimates suggest a seasonal summer CO2 source (Fig. 2). The air–sea CO2 flux of coastal waters is heavily impacted by upwelling (Fig. 3), river plumes, and coastal currents (Ianson et al. 2003; Nemcek et al. 2008; Evans et al. 2012, 2019; Evans and Mathis 2013). Upwelling along the Pacific eastern boundary shelf has contrasting impacts on the oceanic CO2 sink (Fig. 3). Upwelling stimulates biological CO2 uptake by supplying nutrients for primary production (Messié and Chavez 2015) leading to very strong atmospheric uptake values in bloom hotspots (Fig. 3 and Table S1; Ribalet et al. 2010). Upwelling also transports high-CO2 water from depth to the surface, counteracting biological uptake and temperature-driven CO2 uptake (Fig. 3; Christensen 1994; Ianson and Allen 2002; Feely et al. 2008; Chan et al. 2017). The balance of upwelling to downwelling strength has been shown to be a dominant control on air–sea CO2 flux along the Canadian Pacific continental slope and shelf (Ianson et al. 2009). In general, regions further north, such as Queen Charlotte Sound, are expected to act as a stronger atmospheric CO2 sink driven by stronger winter downwelling pushing high-CO2 subsurface shelf waters offshore (Ianson et al. 2009). Within the Salish Sea, Alaska's Inside Passage, and coastal inland fjords, gas fluxes into and out of the ocean are highly episodic and spatially heterogeneous (Jarníková et al. 2022), owing to seasonal freshwater input, high organic matter fluxes, and longer residence times (i.e., nutrient trapping; Jarníková et al. 2022), and the significant variability in tidal mixing throughout the coastal archipelago of British Columbia (Evans et al. 2022).
Fig. 3.
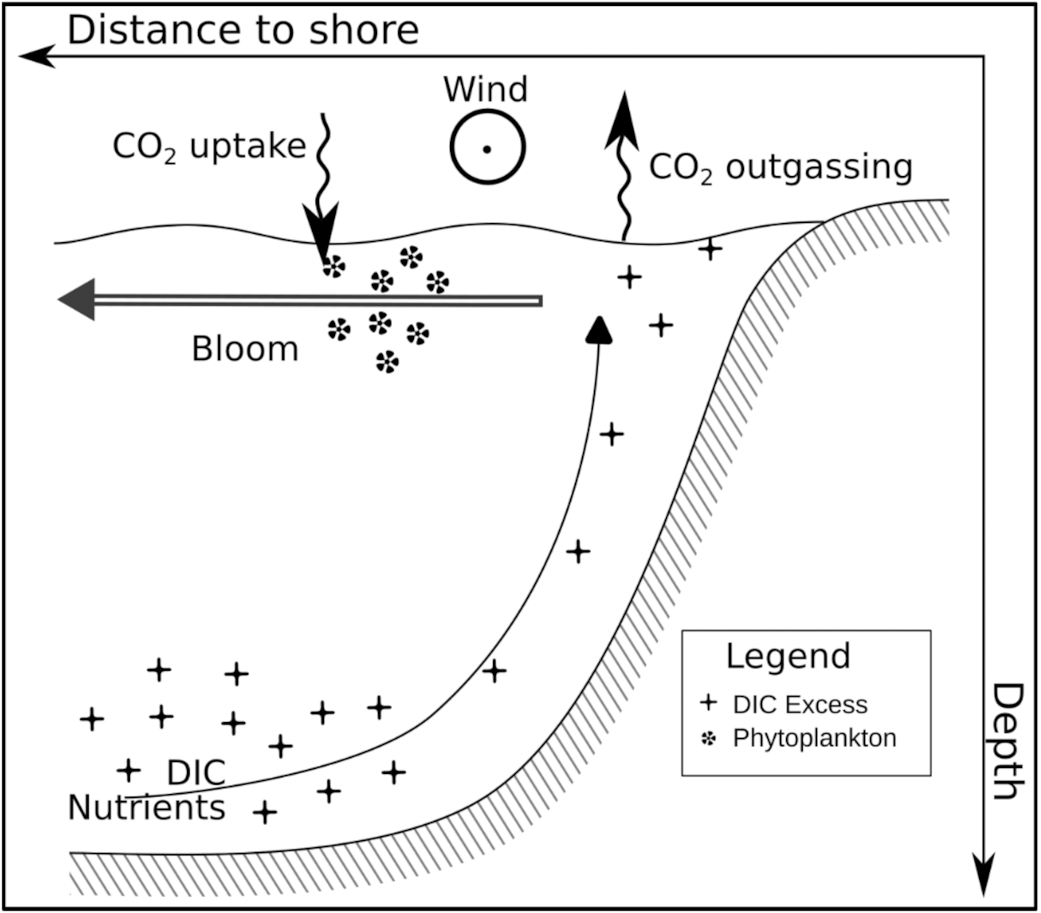
Historically, the Subarctic Northeast Pacific has been relatively well sampled for surface ocean CO2 measurements (Bakker et al. 2016). The Line P program, operated by Fisheries and Oceans Canada, has contributed over 30 years of sustained inorganic carbon system observations. While the program constitutes one of the longest such time series in the global ocean (Freeland 2007; Franco et al. 2021), samples are usually only collected three times a year. The Ocean Station Papa mooring operated by the US-based National Oceanic and Atmospheric Administration offers continuous ocean carbon measurements beginning in 2007 at the oceanic end of Line P (Sutton et al. 2017). Increased international Biogeochemical-Argo profiling float deployments in the region will also likely lead to improved air–sea CO2 flux estimates (Bushinsky et al. 2019). Despite the large number of studies conducted in the Canadian Northeast Pacific (Table S1), the mechanisms driving past and potential future changes in the marine CO2 sink remain unclear (O'Neill et al. 2018). Projected restrictions in upper ocean mixing due to increased seasonal stratification (Durack et al. 2012; Freeland 2013; Cummins and Ross 2020) and warming (Capotondi et al. 2012) will likely alter the seasonal CO2 cycle (Fassbender et al. 2018a, 2018b; Landschützer et al. 2018) and the net flux. The impact of interannual variability (e.g., El Niño-Southern Oscillation, Pacific Decadal Oscillation) and extreme events (e.g., marine heatwaves) on the air–sea CO2 flux is just beginning to be understood (Mogen et al. 2022). Marine heatwaves have already become longer-lasting, more frequent, more extensive, and more intense (Frölicher et al. 2018), with the Northeast Pacific experiencing dramatic temperature anomalies during 2014 and 2019 (Bond et al. 2015; Ross et al. 2019).
Modelling and observation work suggest that the time required to distinguish changes in the magnitude of the ocean carbon sink due to anthropogenic climate change is longer in the Northeast Pacific than in other Canadian ocean basins (McKinley et al. 2016; Sutton et al. 2019; Gooya et al. 2023). The longer time to detection is due to surface ocean CO2 in the region largely increasing at a rate similar to atmospheric CO2. Similar growth rates cause the change in the carbon sink to remain small, while internal variability remains large relative to the anthropogenic signal (Resplandy et al. 2015; McKinley et al. 2016; Sutton et al. 2019). There is a glaring lack of continuous observations during the winter months (the entire region) and year-round in some regions (e.g., coastal waters and regions surrounding Haida Gwaii), which are required to describe this natural variability (Hunter et al. 2015). High-spatial and temporal-resolution regional biogeochemical models have been successful in describing the influence of terrestrial freshwater inputs, spatial heterogeneity in the upwelling zone, and submesoscale eddies (Table S1). However, these modelling studies remain limited in their spatial extent and multiyear coverage required to characterize the entire Canadian West Coast over decadal timescales. Similarly, observations of coastal waters have limited temporal coverage, with most coastal interpolation-based products only capable of producing seasonal climatologies (Table S1).
Enhanced collaboration is needed in improving observational coverage in the Northeast Pacific, communicating with stakeholders (e.g., commercial fishers and aquaculture farmers) and rightsholders (e.g., First Nations) on changing ocean acidification risk, and developing community-first governance policies with respect to ocean CDR approaches. Priority should be placed on building relationships leading to knowledge sharing and knowledge co-production with Indigenous-led groups actively developing and updating marine use plans (Wong et al. 2020). The Marine Plan Partnership Initiative, developed by the province of British Columbia and 17 member First Nations, has already created guiding policy around the management of human activities in northern coastal territorial waters using an ecosystem-based management framework (Marine Planning Partnership Initiative 2015d, 2015b, 2015a, 2015c). The First Nations Health Authority's “We All Take Care of the Harvest” program aims to help coastal communities plan for and manage climate impacts that affect seafood. The Government of Canada's “Salish Sea Initiative” offers funding for collaborative Indigenous marine ecosystem stewardship activities. The First Nations Fisheries Council of British Columbia's action plan is built around relationships and reconciliation, aquatic resource management, safeguarding habitat, and responding to threats like climate change (Haggan et al. 2009; Atlas et al. 2019). Enhanced observation of carbon fluxes and policy around ocean CDR and MRV could be woven into these ecosystem-focused marine use plans and expanded to other communities using the same collaborative framework (Diggon et al. 2021, 2022). This approach would create a strong knowledge base to evaluate climate impacts and ecosystem impacts related to negative emission technologies.
Canadian Atlantic
Overall, the Northwest Atlantic Ocean acts as a net sink of atmospheric CO2 (Fig. 2). However, many coastal regions (e.g., Gulf of St. Lawrence, Scotian Shelf, and Bay of Fundy) potentially act as a source of CO2 for the atmosphere (Fig. 2). The Scotian Shelf, for example, is a highly variable region, with CO2 flux estimates ranging from a strong source of CO2 to the atmosphere (Shadwick et al. 2011; Rutherford et al. 2021) to a weak sink for atmospheric CO2 (Signorini et al. 2013). Air–sea CO2 fluxes in the Labrador Sea are approximately 40% larger relative to those of open ocean regions in the Canadian Pacific, largely due to differences in winter mixing depth between the two regions.
The Labrador Sea is a deep-water formation site where cool, dense water sinks to depth (up to 2000 m in a matter of days; Marshall et al. 1998, 2001) before flowing equatorward as part of the global ocean thermohaline circulation (Wunsch 2002). This process has the potential to move atmospheric CO2 taken up by surface ocean waters to depth (Fig. 4), drawing a direct connection between the atmosphere and the deep ocean, where it can remain trapped for timescales on the order of up to thousands of years (Broecker 1979). Deep water formation renews the region's capacity to maintain high CO2 uptake rates by exposing deep water with little anthropogenic carbon to the atmosphere (Figs. 2 and 4; Gruber et al. 2019a). Given the importance of the Labrador Sea as a region of intense anthropogenic carbon uptake (Khatiwala et al. 2013; Devries 2014; Gruber et al. 2019a), it also represents (at present) one of the largest gaps in CO2 observations in Canadian waters (Table S1). Dominant processes that drive the seasonal variability of surface ocean CO2 in the Central Labrador Sea include deep convection in the fall and winter driven by cooling and by the cyclonic boundary currents in the basin (Fig. 1; Rieck et al. 2019). Deep convection brings high-CO2 and nutrient-rich water from depth to the surface, reducing oceanic uptake of atmospheric CO2 over the winter months (Fig. 4). In the spring, biological uptake acts to reduce CO2 at the surface and increase air–sea CO2 fluxes, leading to enhanced uptake of atmospheric CO2 from spring to summer (Fig. 4; DeGrandpre et al. 2006; Körtzinger et al. 2008; Atamanchuk et al. 2020). Preconditioned by upwelled nutrients driven by the winter deep convection and the increasing supply of sunlight, the North Atlantic spring phytoplankton bloom in the Labrador Sea is one of the most efficient biological carbon pumps globally (Baker et al. 2022). Following the bloom, large organic particles and aggregates sink out of the surface mixed layer due to gravity, moving CO2 to depth (Briggs et al. 2011; Villa-Alfageme et al. 2016). Smaller particles and dissolved carbon are removed from the surface in the fall and winter by vertical mixing through deepening of the surface mixed layer (Dall'Olmo et al. 2016; Lacour et al. 2019), eddy activity (Resplandy et al. 2019), and large-scale subduction (Hansell et al. 2009). The succession of these carbon export fluxes in the Labrador Sea allows continuous carbon export to depth year-round (Fig. 4; Boyd et al. 2019). Over interannual and decadal timescales, during the positive phase of the North Atlantic Oscillation, subpolar regions experience increased vertical mixing and lower sea surface temperatures, driving variability in Northwestern Atlantic Ocean CO2 fluxes (Thomas et al. 2008; Ullman et al. 2009; Yashayaev and Loder 2017). Under global warming, shoaling of the mixed layer depth and the addition of glacial meltwater could impact the future biological regime of the Labrador Sea by increasing stratification (Balaguru et al. 2018; von Appen et al. 2021).
Fig. 4.
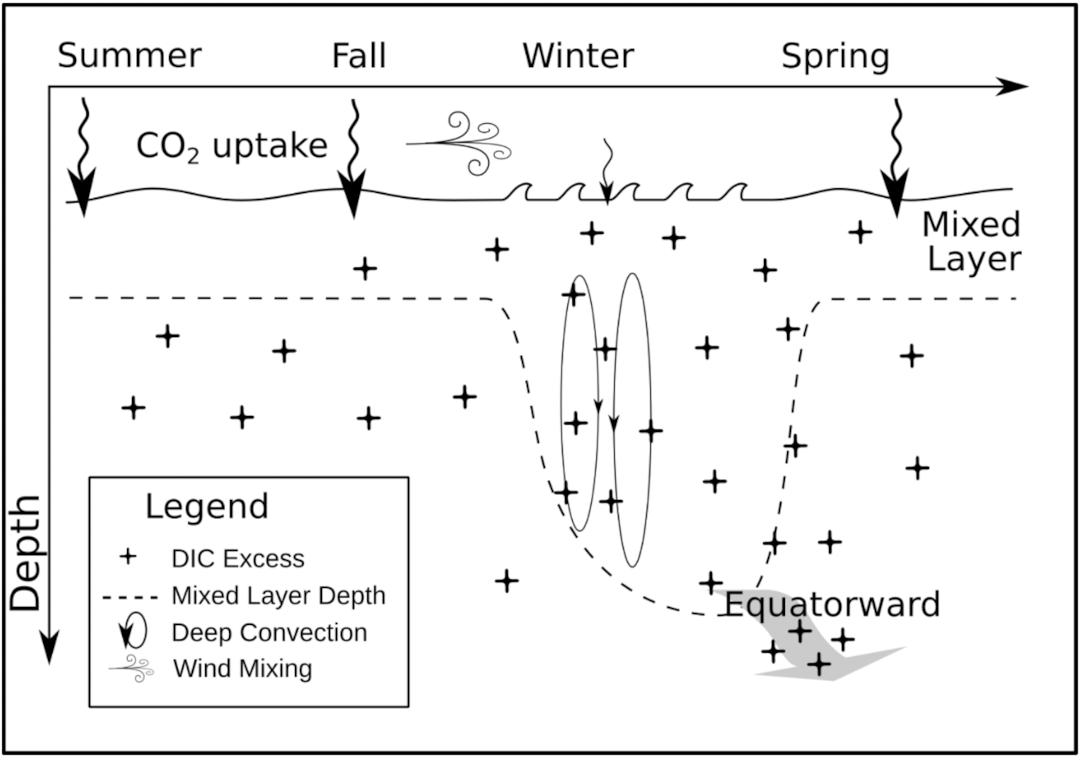
As the cold and fresh Labrador Sea current warms flowing southward along the Newfoundland-Labrador and Scotian Shelf (Fig. 1), surface ocean CO2 levels rise above saturation, leading to outgassing, uncharacteristic of high-latitude continental shelves (Fig. 2). This coastal environment is further complicated by the upwelling of cold, high-CO2 and nutrient-rich waters along the continental slope, which increase surface ocean CO2 levels to feed the spring phytoplankton bloom before reducing CO2 again. This process is comparable to upwelling in the Northeast Pacific (Fig. 3). However, unlike the West Coast of Vancouver Island, where the high-CO2 upwelled water causes an increase in surface ocean CO2 (Evans et al. 2012), on the Scotian Shelf, the cooling effect of the upwelled water has been shown to overwhelm the CO2 increase, resulting in a net decrease in surface ocean CO2 (Rutherford et al. 2021). The reason for this difference is linked to global ocean circulation patterns, where subsurface waters in the Pacific tend to accumulate a greater amount of DIC due to the remineralization of organic matter (England 1995). The competing mechanisms of upwelled water on air–sea CO2 fluxes are highly variable spatially and temporally, making them difficult to capture in observations or numerical models. This difficulty can lead to diverging results between studies characterizing the Scotian Shelf as both a source and sink of CO2 (e.g., Table S1; Shadwick et al. 2011; Laruelle et al. 2014; Rutherford et al. 2021). In the Gulf of St. Lawrence, tidal and estuarine mixing brings respired organic matter into the surface layer, driving CO2 outgassing in the shallow mouth of the estuary (Dinauer and Mucci 2017). In the deeper oceanward part of the St. Lawrence region, enhanced biological drawdown keeps surface ocean CO2 undersaturated, driving net uptake from the atmosphere (Dinauer and Mucci 2017). However, these fluxes may shift in the future due to increased biological production (Dinauer and Mucci 2018).
Air–sea CO2 fluxes at both offshore and coastal regions of the Canadian Northwest Atlantic are directly affected by the formation of Labrador Sea Water, which in turn drives the variability and intensity of the Labrador current. Uncertainty in air–sea CO2 fluxes is largely attributed to the sparsity of direct observations in the Labrador Sea and much of the subpolar North Atlantic, a lack of agreement between wind speed products (Atamanchuk et al. 2020), and not enough direct estimates of the volume of Labrador Sea water formation during any given winter (Li and Lozier 2018). Most surface ocean CO2 observational data in the Northwest Atlantic Ocean comes from the Ship-Of-Opportunity Program using volunteer merchant ships, with spatial coverage most densely concentrated around busy shipping tracks (Bakker et al. 2016). Regions of greatest spatial coverage include the Southern Labrador Sea, the Gulf of Maine, and the Gulf Stream region south of Nova Scotia off the east coast of the United States (Fig. 1), whereas the Grand Banks region, along with both the shelves of Labrador and Newfoundland, as well as the Central Labrador Sea remain very data sparse. For those data-poor regions, ongoing monitoring programs like the Atlantic Zone Off-Shelf Monitoring Program and Atlantic Zone Monitoring Program (Therriault et al. 1998; Ringuette et al. 2022) are making important efforts to provide additional continuous observations, but are still limited to summer sampling programs. International monitoring programs also contribute significantly to observations in the region, such as GO-SHIP (AR07W, A02, and Davis monitoring lines), Overturning in the Subpolar North Atlantic Program (OSNAP), and Biogeochemical-Argo (Lacour et al. 2019). The absence of buoys measuring wind speed in the Labrador Sea also contributes to air–sea gas exchange uncertainties (Atamanchuk et al. 2020). Improving our understanding of the controls on air–sea CO2 fluxes in the Central Labrador Sea may even lead to improved estimates for the whole North Atlantic basin (Friedrich and Oschlies 2009). The importance of the region for global marine carbon uptake emphasises the value of maintaining the Atlantic Repeat Hydrography Line AR07W line operated by Fisheries and Oceans Canada (Hall et al. 2013) across the Central Labrador Sea. The use of new autonomous sensing platforms for measuring CO2 (e.g., using wave gliders as in DeYoung et al. 2020) may also play an important role in gap filling. The success of a few previously deployed long-term moorings in the region has greatly improved our understanding of the seasonality of CO2 fluxes in the Labrador Sea, such as the most recent completed by the SeaCycler deployment in 2016/2017 (Atamanchuk et al. 2020) and the others deployed in the early 2000s (DeGrandpre et al. 2006; Körtzinger et al. 2008; Martz et al. 2009).
Like in Pacific Canada, traditional knowledge exchange and collaboration between the scientific community, government entities, and First Nations can prove to be extremely successful. Atlantic Canada is home to many Indigenous groups, offering immense opportunity for traditional knowledge exchange and collaboration in ocean observing/monitoring efforts (Proulx et al. 2021). Alexander et al. (2019) mapped past research (in marine management, monitoring, and marine research) published in collaboration with Indigenous communities in Canada. In Atlantic Canada, only five case studies were found in the literature, making this region the one with the fewest collaborations compared with the Arctic and Pacific Canada. Further, in the report of Moran et al. (2022), there is only one community monitoring platform directly collaborating with Indigenous groups on the East Coast, located in Placentia Bay, Newfoundland. Yet, Eger et al. (2021) show increasing opportunities for integrated marine management with Indigenous groups in the Bay of Fundy area. While historically, Atlantic Canada has missed a myriad of opportunities within these Indigenous collaborations, there is a promising new Atlantic Regional Association of the Canadian Integrated Ocean Observing System—CIOOS (Stewart et al. 2019) pushing to create programs with Indigenous communities (Proulx et al. 2021). As early career ocean professionals (ECOPs), we strongly suggest that the efforts of CIOOS-Atlantic include air–sea CO2 fluxes as a research area of focus in both coastal and offshore regions of Atlantic Canada.
Canadian Arctic
The Arctic Ocean is predominantly a CO2 sink (Fig. 2). Current estimates indicate that the pan-Arctic Ocean constitutes 5% to 14% of the global oceanic CO2 uptake, despite covering only 3% of the global ocean area (Bates and Mathis 2009). Uptake values reported in the Canadian Arctic are among the highest in Canadian waters (Fig. 2), but are sparse and highly disparate in space and time (Table S1).
Arctic Ocean air–sea CO2 fluxes are uniquely impacted by the presence of sea ice, which effectively prevents air–sea CO2 exchange (Figs. 1 and 5). Variability in ice conditions thus explains part of the regional and temporal distribution of CO2 uptake, with areas of longer open water seasons being stronger sinks (e.g., Chukchi Sea, Baffin Bay, Labrador Sea; Fig. 2). Harsh Arctic weather and ice conditions induce a seasonal bias in field observations, restricting most scientific campaigns to take place over the summer months (Table S1). This observation gap is usually waived by considering non-open water seasons as negligible to the annual contribution (e.g., Loose et al. 2011; Ahmed et al. 2021). However, observed wintertime CO2 fluxes in comparatively smaller scale polynyas and ice leads are one to two orders of magnitude higher than predicted by theory in open waters (Else et al. 2011). On top of the physical flux barrier, sea ice chemistry affects CO2 fluxes through the sea ice carbon pump during both sea ice formation and melt (Rysgaard et al. 2011). During sea ice melt, dissolution of ikaite (a form of calcium carbonate in sea ice) lowers surface ocean CO2, increasing the potential for atmospheric uptake (Fig. 5; Rysgaard et al. 2013). During sea ice formation, high-CO2 brine within the ice is rejected into the underlying seawater and sinks to depth (Fig. 5; Rysgaard et al. 2007). Both processes significantly modify air–sea CO2 fluxes during fall and spring (DeGrandpre et al. 2019; Mortenson et al. 2020; Duke et al. 2021). Finally, sea ice also impacts biological CO2 drawdown in a number of ways, by imparting local controls on the timing, duration, and magnitude of spring and summer primary production (Rysgaard et al. 1999; Arrigo et al. 2008; Søreide et al. 2010; Arrigo et al. 2012).
Fig. 5.
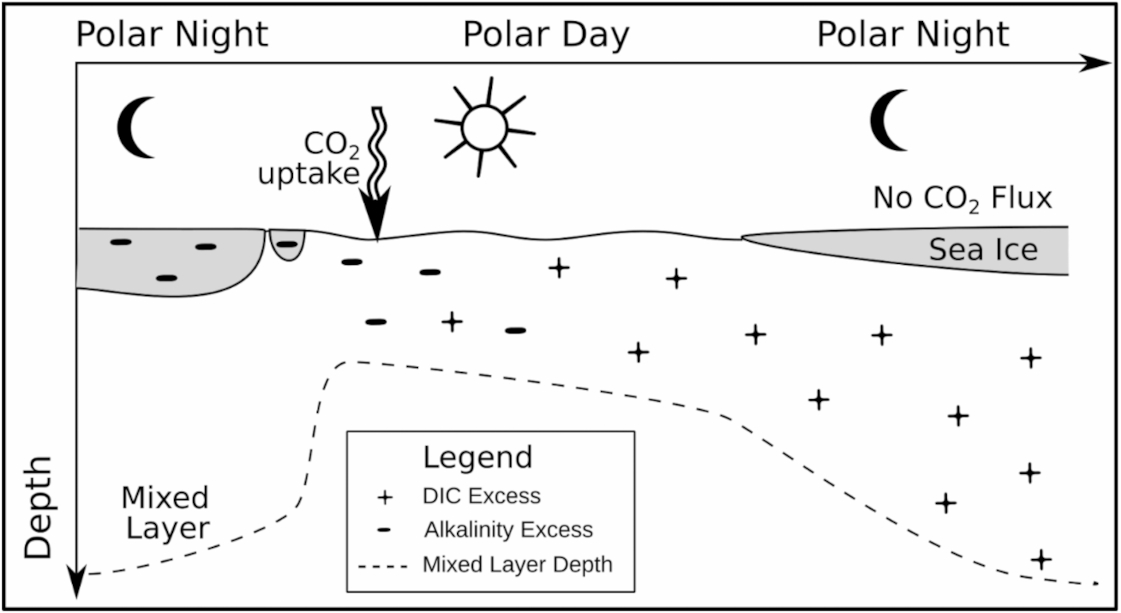
Regardless of the past or present state of the carbon budget in the Arctic Ocean, its future is likely to be significantly different. The Arctic is warming at two to three times the rate of global warming (IPCC 2019). Sea ice decline is the archetype of climate change signals. The reduction of multi-year sea ice over the last decade has resulted in large portions of the Canadian Arctic becoming seasonally ice-free (Yamamoto‐Kawai et al. 2009; Laxon et al. 2013; Wang et al. 2018) and a lengthening of the open water season (Maslanik et al. 2007; Perovich et al. 2007). As a result, the ocean surface is becoming increasingly exposed to atmospheric CO2 uptake (DeGrandpre et al. 2020). In fact, Ahmed and Else (2019) estimated an increase in CO2 uptake in the Canadian Arctic Archipelago in the last four decades associated with increased sea ice loss and higher wind speeds. While a longer ice-free season introduces greater light availability for primary production, nutrient replenishment of surface layers could either be enhanced by increased wind mixing or reduced by increased stratification due to ice melt (Lannuzel et al. 2020). Therefore, the evolution and impact of primary production on the Arctic marine carbon cycle remain an open question (Arrigo et al. 2008; Tremblay et al. 2015; Lannuzel et al. 2020). Meanwhile, changes to the upper ocean's salinity and temperature structure in the Barents and Kara Seas, referred to as Arctic “Atlantification” (e.g., Årthun et al. 2019), may introduce potential consequences on ice formation and deep convection in the Eurasian Basins (Timmermans and Marshall 2020). Moreover, coastal margins and the ice edge can also be important pathways for carbon export (Nishino et al. 2011), particularly as phytoplankton blooms may be stimulated by nutrients derived from coastal rivers or seeded by ice algae (Matthes et al. 2021). There is, however, some evidence that rivers may drive localized marine organic carbon respiration (Izett et al. 2022), suggesting that some Arctic regions may experience periodic net CO2 outgassing (Manning et al. 2020). Finally, it is likely that future Arctic CO2 fluxes will exhibit varied responses to ongoing permafrost thawing and changes in river runoff, through various effects on nutrient and organic matter inputs, and changes to local marine stratification (Vonk and Gustafsson 2013; Prowse et al. 2015). Further, increased methane bubbling and hydrate erosion will affect the system (Westbrook et al. 2009).
In the context of this evolving Arctic Ocean with more mobile ice packs, new ice-proof, autonomous observing technologies are needed to close the fall, winter, and spring observational gaps. Those technologies already exist in the form of Ice-Tethered Profilers and Arctic Ocean Flux Buoys, capable of year-round measurements, and regularly deployed in the Beaufort Gyre. Meanwhile, renewed efforts to install and maintain eddy covariance instruments in Iqaluktuttiaq (
, Cambridge Bay; Butterworth and Else 2018) or to expand the Barrow Strait Real Time Observatory will be instrumental in providing year-round observations. In addition to these local observations, international scientific partnerships targeting a pan-Arctic approach will be crucial to addressing the relevant questions surrounding the quantification of CO2 fluxes in the Arctic Ocean. Examples include the Synoptic Arctic Survey (Paasche et al. 2019), the Pacific Arctic Group, the Distributed Biological Observatory (Moore and Grebmeier 2018), and Ecosystem Studies of Sub-Arctic and Arctic Seas (ESSAS). The use of numerical models, with a coupled sea ice biogeochemistry component covering the Arctic Ocean, will also be important to obtain a more comprehensive understanding of the carbon system in the northernmost Canadian Ocean. The future fate of the Arctic Ocean atmospheric CO2 sink could “possibly increase or decrease”, as detailed in Lannuzel et al. (2020). Such high uncertainty is intrinsically linked to the high complexity of the Arctic carbon cycle and to the drastic environmental changes currently unfolding in that region. Enhanced efforts are required to better observe and understand this high complexity and to anticipate those drastic changes.

Scientific research conducted in the Canadian Arctic has historically been motivated, designed, and implemented from a southern settler perspective. This results in Inuit Nunangat people being excluded and marginalized from the benefits of northern research. Inuit Tapiriit Kanatami (2018) provides vision and strategy implementation for empowering research in Inuit Nunangat (i.e., the Inuvialuit Settlement Region (Northwest Territories), Nunavut, Nunavik (Northern Québec), and Nunatsiavut (Northern Labrador)) at a national level. Pedersen et al. (2020) share 45 recommendations developed by Ikaarvik (meaning “bridge” in Inuktitut) youth and mentors for researchers aiming to meaningfully consult, engage, and incorporate Inuit communities in scientific research. This work builds on the concept of ScIQ, the combination of Inuit Qaujimajatuqangit (IQ) and science. Pedersen et al. (2020) describe Inuit Qaujimajatuqangit as a way of knowing and a way of life that extends beyond traditional knowledge, including knowledge, customs, and values, encompassing relationships, attitudes, and behaviours. The recommendations describe actions researchers can take before, during, and after conducting research in the north to incorporate Inuit Qaujimajatuqangit within the entirety of the scientific process.
Research gaps
There are still prominent gaps in our understanding of air–sea CO2 flux variability across the Pacific, Atlantic, and Arctic Oceans (Table 1). Scientific efforts in Canada and through international collaborations in both observations and modelling have narrowed the uncertainties associated with specific basin air–sea CO2 fluxes (Fig. 2 and Table S1). However, these efforts are largely focused on resolving variability in the seasonal cycle and determining mean annual flux values. We still severely lack understanding of how air–sea CO2 flux variability is impacted on longer timescales or how fluxes may be shifting under climate change (Table 1). Given the different processes that dominate spatial and temporal heterogeneity in air–sea CO2 fluxes (Figs. 3–5), we have summarized the major basin-specific, process-focused research questions needed to advance the field (Table 1).
Table 1.
Gap | Research question |
---|---|
Shared across basins | |
Long-term change | Where and why is the surface ocean increase in CO2 different from the atmospheric trend, altering the ocean carbon sink? |
Sub-decadal to decadal variability | How do modes of climate variability impact air–sea CO2 fluxes (e.g., El Niño-Southern Oscillation, Pacific Decadal Oscillation, North Atlantic Oscillation)? |
Ocean carbon dioxide removal verification | How can ocean carbon dioxide (CDR) interventions be differentiated from signals of natural variability and anthropogenic climate change? |
International collaboration | Where can Canadian observation and modelling efforts collaborate with, benefit from, and contribute to international ocean understanding? |
Indigenous partnerships and capacity building | How can research funding be leveraged to enhance collaboration with First Nations to increase observations, address impending ocean CDR monitoring, reporting, and verification (MRV) development, and ocean acidification action planning? |
Complete marine carbon budgets | What is the complete marine carbon budget in Canada, integrating carbon stocks and fluxes across other marine pools (e.g., pelagic, benthic, and terrestrial to marine interface)? How can we quantify marine carbon budgets and rates of change in more variable and dynamic regions (e.g., nearshore, upwelling regions, polynya regions, etc.)? How can we combine different observation types (e.g., discrete, underway, autonomous, observation-based products) and model outputs to resolve “policymaker-relevant” flux values (g C year−1)? |
Pacific Ocean | |
Marine heatwaves | How will future longer-lasting, more frequent, and more intense marine heatwaves change regional air–sea CO2 fluxes? How will these impact primary productivity in the iron-limited, high-nutrient low-chlorophyll region? |
Upwelling to downwelling strength | What is the balance of upwelling to downwelling strength that differentiates net annual uptake from outgassing? How is this pattern distributed spatially along the Canadian West Coast? |
Atlantic Ocean | |
Deep-water formation rates | How is climate change impacting Labrador Sea deep-water formation rates and depths? How is carbon storage durability being impacted? |
Biological carbon pump | How will shoaling winter mixed layer depths under climate change impact phytoplankton spring blooms and dissolved inorganic carbon cycling? |
Scotian Shelf processes | What is the net impact of upwelling on surface ocean CO2? How do phytoplankton bloom initiation timing and spatial distribution change the net annual flux on the Shelf? |
Arctic Ocean | |
Sea ice changes | How is a younger, thinner sea ice cover with a smaller spatial extent and a longer open water season changing the sign/magnitude of air–sea CO2 fluxes? How is this changing sea ice carbon pump dynamics? |
Freshwater stratification and productivity | How will changes in surface stratification from sea ice melt, increased glacial runoff, and changes in terrestrial runoff (e.g., permafrost thaw and riverine input) impact air–sea CO2 fluxes due to differing water mass carbon loads and equilibration time? How will this impact the timing and magnitude of phytoplankton blooms? |
Future directions
The next generation of oceanographers is witnessing the emergence of a new ocean state. The need to reduce present-day uncertainties, enhance our understanding of tipping points, account for extreme climatic events in the ocean, and document change from the preindustrial baseline state presents exciting challenges for the oceanographic community. These challenges are particularly relevant to understanding air–sea CO2 fluxes across all three of Canada's adjacent ocean basins. Expanded use of emerging techniques and greater cross-collaboration between observation and modelling specialists could narrow the range of uncertainty in regional to basin-scale fluxes, improve observational coverage, inform carbon stocktake efforts, establish a baseline for proposed ocean CDR projects, and support ocean acidification mitigation and adaptation efforts (Table 2).
Table 2.
Category | Recommendations | Examples |
---|---|---|
Observations | 1. Expand use of innovative autonomous measurement technology and support its development. 2. Submit relevant data to public repositories and invest in maintaining global data repositories/structures (national and international). 3. Integrate multiple knowledge systems. | • Instrument platforms (e.g., gliders, surface vehicles, deep-water floats, profiling moorings, and ice-proof platforms) • Sensors (e.g., mobile high frequency dissolved inorganic carbon and total alkalinity, and pCO2 remote sensing capabilities) • Satellite remote sensing • Repositories (e.g., Canadian Integrated Ocean Observing System (CIOOS), Surface Ocean CO2 Atlas (SOCAT), and Global Ocean Data Analysis Project (GLODAP)) • Mine data so earlier data are not lost • CARE/FAIR data sharing principles • Alternative data sources (e.g., qualitative data) • Contextualizing Western science data |
Modelling | 4. Direct modelling efforts towards fit-for-purpose ocean CDR and regional process study applications. | • Observation data assimilation and evaluation • Multi-model ensemble comparison projects • Regional downscaling • Use of emergent constraint techniques |
Ocean carbon dioxide removal | 5. Develop transparent and robust monitoring, verification, and reporting (MRV) protocols. 6. Engage and consult with Indigenous communities. 7. Mobilize ocean acidification expertise. | • Clearly distinguishing intervention from baseline noise • Integration of observations and models • Establish code of conduct • Adherence to community specific needs (e.g., First Nations marine governance) • Canada's Ocean Acidification Community of Practice, Ocean Acidification International Coordination Centre, Global Ocean Acidification Observing Network, and NOAA Joint Ocean Acidification Framework |
Indigenous co-generation of knowledge | 8. Tailor community-specific approaches. | • Formulate research questions through community collaboration • Pre-study engagement and frequent collaboration thereafter • Collaborations built on meaningfully trusting relationships • Participation beyond data collection |
Early career capacity building | 9. Increase significance of meaningfully engaging Indigenous communities. 10. House specific projects in multidisciplinary collaborative platforms. 11. Improve equity, diversity, and inclusion. | • Required course work on Indigenous history and rights • Contribute to established community relationship continuity • Recognize community building activities within dissertations • Collaborate with interdisciplinary researchers to deliver improved community-centered outcomes • Expanded recruitment to include traditionally marginalized groups valuing nontraditional metrics of success • Fair and equitable financial support for graduate students and postdocs |
Maturing autonomous carbon system sensor technology (Sonnichsen et al. 2023) and deployment on innovative autonomous monitoring platforms such as gliders, surface vehicles, floats, and profiling moorings offer increased observational capacity beyond time series and sporadic underway sampling (Sastri et al. 2019; Chai et al. 2020). New and planned satellite missions offer improved observation capabilities, particularly of the active gas exchange surface layer (Woolf et al. 2016; Watson et al. 2020) and of surface and vertical water transport (Ardhuin et al. 2018; Oubanas et al. 2018), enabling measurement of biogeochemical fronts associated with upwelling, marginal sea-ice zones, and across heterogeneous continental shelf boundaries and river outflows (Shutler et al. 2020). Furthermore, submission of surface ocean CO2 observation data to global databases (e.g., Surface Ocean CO2 Atlas; Bakker et al. 2016) is extremely important to increase accessibility, quality assurance, and control of data, as well as end-user reusability. The principles of FAIR (Findable, Accessible, Interoperable, and Reusable; Tanhua et al. 2019) and CARE (Collective Benefit, Authority to Control, Responsibility, and Ethics; Tanhua et al. 2019; when relevant using Indigenous-owned data and knowledge) should be adhered to when considering a project's data lifecycle. These breakthroughs in innovative observation platforms and increasing public availability of data coincide with the emergence of machine learning and higher computing capacity that can be used to simulate the marine carbon system during periods or within regions devoid of sufficient observations (Landschützer et al. 2014) or to project future changes. Integrating multiple ways of knowing outside conventional western science observations can result in richer outcomes with greater breadth from a stronger framework of research questions established through early engagement (Ban et al. 2018). Indigenous peoples’ communal memory, as an example, is capable of observing trends or variations in their lands that no other sensor can replicate (Alessa et al. 2016), often outside western science monitoring metrics (Table 2). This could include contributing alternative data sources (e.g., qualitative measures embedded in traditional laws or stories; Ban et al. 2018) or contextualizing, interpreting, and applying results from earth observations (e.g., Mittimatalik sea ice charts; Wilson et al. 2021).
Considering, specifically, the marine carbonate system, existing numerical models need to be carefully calibrated against observations, and parameterizations need to be improved. Observations are needed to evaluate the performance of existing models and carefully calibrate them through data assimilation to narrow the spread of air–sea CO2 flux estimates across model ensembles (Wang et al. 2016). Assimilation of observations, especially biogeochemical data, will improve understanding of historical carbon uptake conditions and drivers of variability. Data assimilation also improves near-real-time seasonal to decadal predictions (forecasts), which are currently only indirectly initialized (Li et al. 2019). Improved observational coverage, for example by autonomous biogeochemical ocean Argo floats, will improve our ocean modelling ability. Idealized model experiments like those in Sarmiento et al. (1998) and Winton et al. (2013) and multimodel ensemble comparison projects like those in Cheng et al. (2013) and Frölicher et al. (2015) can be used to understand the relative importance of different biogeochemical processes and their response to the changing climate. Further, these types of experiments can be important for identifying the source of model ensemble uncertainty. Model uncertainty in the ocean carbon flux is projected to be largest where surface waters are connected to deeper waters (Gooya et al. 2023). Improving ocean circulation in models, which is a primary driver of ocean carbon flux variability (McKinley et al. 2020), can reduce these uncertainties. Regional downscaling of low-resolution models to higher resolution, especially in heterogeneous regions like the Canadian Arctic Archipelago, can result in more informative model projections (Table 2). As an example, mesoscale eddies are quite important for mixing (and therefore also impact air–sea CO2 fluxes; Ford et al. 2022), but are often not resolved in current generations of earth system models (Frölicher et al. 2015). Further, simplified and specialized models can analyze the efficiency and climate-level feedback of various proposed ocean CDR techniques. “Sampling” from models (looking at data from where and when we have real-world observations within the full model field) can be used to evaluate the performance of current observation gap-filling techniques (Gloege et al. 2021) in regions of high air–sea CO2 fluxes and high uncertainty (e.g., high-latitude oceans; Gruber et al. 2019b). Moreover, new statistical tools and techniques such as emergent constraints (a way of looking at the relationship between a variable of current climate state within individual models, and future changes in a variable of interest that make up an ensemble) accelerate the development and improvement of the next generations of earth system models (e.g., Hall et al. 2019; Bourgeois et al. 2022).
Our poor understanding of air–sea CO2 flux variability represents a major gap in current ocean CDR and carbon credit generation program standards (Tables 1 and 2). Negative emission technologies must be additional to what would have happened by law or under a business-as-usual scenario if the project had not been carried out (Verra 2022). Enhanced capacity and accuracy in both observations and modelling efforts mentioned above can reduce air–sea CO2 flux uncertainty, which is critical to clarifying what constitutes additional removal relative to baseline noise. However, as far as developing trusted, unique, nonexchangeable carbon credits from nature-based, mechanical, or geoengineered solutions (NASEM 2021), considerations need to be made for which carbon pool is being drawn down. Accounting must include the transboundary nature of the ocean, the timescale of carbon removal, and, most importantly, if the process actually enhances ocean atmospheric CO2 uptake. We are much further behind in defining the marine carbon stocktake compared to the terrestrial carbon reservoir in Canada (Sothe et al. 2022). Moving forward with marine nature-based solutions that include tangible ecosystem co-benefits (e.g., ocean acidification mitigation) through restoration and conservation should continue to be a priority while recognizing their limitations and potential leakage (Drever et al. 2021; Williamson et al. 2022; Roth et al. 2023). Considerations also need to be given to ensuring the safety and efficacy of ocean CDR given the risk of uncertain impacts on human and environmental welfare through a comprehensive code of conduct (Loomis et al. 2022). Ocean CDR projects need to concentrate on acquiring funding at the levels highlighted in the NASEM (2021) report and conducting feasibility and scalability testing with a focus on monitoring, reporting, and verification. The latter should be performed through a lens of governance in line with equity and justice goals (Kosar and Suarez 2021; Loomis et al. 2022). Ocean CDR should not be used to delay carbon emission reductions (Shutler 2020; Ho 2023).
Resolving air–sea CO2 fluxes helps resolve uncertainty in ocean acidification, as strong atmospheric CO2 uptake generally leads to elevated trends and worsening ocean acidification conditions. Leveraging existing ocean acidification infrastructure, expertise, and policies offers an exceptional starting point for addressing uncertainty in air–sea CO2 fluxes and developing ocean CDR MRV (Table 2). National and international ocean acidification infrastructure already exists (e.g., Canada's Ocean Acidification Community of Practice; Ocean Acidification International Coordination Centre; Hansson et al. 2014; Global Ocean Acidification Observing Network; Newton et al. 2015; DFO-NOAA Joint Ocean Acidification Framework; Government of Canada and Fisheries and Oceans Canada 2018), along with widespread public attention (United Nations Sustainable Development Goal 14.3; Barbière et al. 2019). Experts from these communities are well suited to address monitoring gaps in air–sea CO2 flux observations, assess ocean CDR ecosystem impacts, and offer the public a trusted voice advancing MRV development.
Throughout this paper, we have identified Indigenous-led or co-led monitoring programs and coast-specific Indigenous scientific collaborative frameworks built on recommendations from First Nations. Indigenous communities are likely to experience greater climate impacts in Canada, while their contribution to the global climate crisis is negligible. Indigenous peoples are a highly sensitive population at the intersection of climate change and community health (Ford et al. 2018; Kenny et al. 2020), facing a burden of existing social disparity in health, education, food and energy security, generational trauma, and colonial legacies (Ford and Smit 2004; Ford et al. 2010; Maldonado et al. 2013; Maru et al. 2014). With an elevated emphasis from research and government institutions on meaningfully engaging with First Nations, new collaborations could improve traditional knowledge exchange to enhance marine carbon cycle understanding. The community-specific approach would follow successes in mapping (Davies et al. 2020; Bishop et al. 2022), coastal management (Weiss et al. 2013; Lombard et al. 2019), marine conservation (Ban et al. 2009), observational oceanography (Moran et al. 2022), and fisheries (Weatherdon et al. 2016; Turgeon et al. 2018; Reid et al. 2021). As ocean CDR and Indigenous involvement in the sector are both just emerging, any new collaborative initiative should follow recommendations made by Breckwoldt et al. (2021), including (1) the need for participation beyond data collection, (2) acknowledgment and mitigation of an agenda mismatch between funded and needed research, and (3) emphasizing the power of the transdisciplinary processes of learning together.
Pathways for early career researchers to meaningfully engage with Indigenous groups and collaborate on climate problems are restricted by institutional undervaluing, graduate student timelines, a lack of funding, and traditional academic metrics of success (e.g., peer-reviewed journal publications). University students, and particularly international students, may lack knowledge about Canada's colonial history and systemic oppression of Indigenous peoples (Godlewska et al. 2020) and the ways that natural science research can impact Indigenous communities (Bozhkov et al. 2020; Kater 2022). Community relationship building needs to be recognized as a priority investment and should start with mandatory course work on Indigenous history and rights taught by Indigenous instructors to enhance student understanding of the socio-political landscape around their research (Table 2; Wong et al. 2020). Given graduate student timelines, it falls on the principal investigators to identify which Indigenous government or community has jurisdiction over or interests in the proposed research. Principal investigators can create continuity in community relationship building, which is critical to establishing trust and genuinely engaging with rightsholders (Table 2). Early dialogue should support Indigenous peoples’ self-determination, focusing on what research is being proposed and how the proposal meets the interests and priorities of Indigenous communities while finding opportunities for reciprocity (Wong et al. 2020). Mainstreaming reconciliation in all aspects of the scientific endeavour, from formulation to completion, as a requirement in Government of Canada tri-council funding (Wong et al. 2020), integrated as a valued component of traditional graduate student dissertations, and moving forward with both treaty-based and resurgence-based decolonial Indigenization of academic spaces and places is severely overdue (Gaudry and Lorenz 2018).
Training and equipping ECOPs with the skills needed to apply the approaches described above should be a priority moving forward in supervised academic settings as well as in government and industry work environments. Early exposure to carbon cycle concepts, interdisciplinary linkages, and skill building through undergraduate research assistantships is ideal if accompanied with adequate compensation and professional development opportunities. Early career researchers should not be expected to become experts in all the methods outlined throughout this paper, including community collaboration and engagement (Table 2). Rather, early career researchers should be given the opportunity to connect (as part of their research project) to a platform that enables them to collaborate with other multidisciplinary researchers, bringing together social scientists, economists, and Indigenous knowledge keepers. Beyond training, at the forefront of recruiting students all the way to research chairs, the focus should be on increasing equity, diversity, and inclusion within our field to spark new ideas, solutions, and perspectives (Osiecka et al. 2022). Fair and equitable financial support for graduate student and postdoc work (Laframboise et al. 2023), mental health support, and fostering greater peer-to-peer collaborative opportunities lead to more diverse, happier, healthier, and more productive labs (Osiecka et al. 2022). The next generation of ocean scientists faces significant adversity in informing policy efforts to meet global net-zero emissions targets while grappling with past and current injustices around truth and reconciliation efforts here in Canada. Among this group of ECOPs, there is consensus on the need for recentering science in future policy discussions while moving forward with all available options to combat the climate crisis.
Acknowledgements
Heartfelt thanks to Roberta Hamme for her passion, enthusiasm, and support for all the early career highly qualified personnel on Canada's Marine Carbon Sink Project. We are so grateful to have so much support for our ongoing work from all the principal investigators on the project, including Debby Ianson, Brent Else, Philippe Tortell, Doug Wallace, Paul Myers, Kent Moore, Katja Fennel, Brad deYoung, Dariia Atamanchuk, Frédéric Cyr, Susan Allen, Nadja Steiner, Neil Swart, Lisa Miller, Kumiko Azetsu-Scott, Jim Christian, Emmanuel Devred, Youyu Lu, Tetjana Ross, and Igor Yashayaev. We also thank fellow early career ocean carbon professionals Robert Izett, Tereza Jarníková, and Samantha Jones for their support and feedback while putting this manuscript together, as well as the two anonymous reviewers for their helpful comments.
References
Ahmed M., Else B.G.T. 2019. The ocean CO2 sink in the Canadian Arctic Archipelago: a present-day budget and past trends due to climate change. Geophys. Res. Lett. 46.
Ahmed M.M.M., Else B.G.T., Butterworth B., Capelle D.W., Guéguen C., Miller L.A., et al. 2021. Widespread surface water pCO2 undersaturation during ice-melt season in an Arctic continental shelf sea (Hudson Bay, Canada). Elementa, 9(1): 1–22.
Alessa L., Kliskey A., Gamble J., Fidel M., Beaujean G., Gosz J. 2016. The role of Indigenous science and local knowledge in integrated observing systems: moving toward adaptive capacity indices and early warning systems. Sustain. Sci. 11(1): 91–102.
Alexander S.M., Provencher J.F., Henri D.A., Taylor J.J., Lloren J.I., Nanayakkara L., et al. 2019. Bridging Indigenous and science-based knowledge in coastal and marine research, monitoring, and management in Canada. Environ. Evid. 8(1): 1–24.
von Appen W.-.J., Waite A.M., Bergmann M., Bienhold C., Boebel O., Bracher A., et al. 2021. Sea-ice derived meltwater stratification slows the biological carbon pump: results from continuous observations. Nat. Commun. 12(1): 1–16.
Ardhuin F., Aksenov Y., Benetazzo A., Bertino L., Brandt P., Caubet E., et al. 2018. Measuring currents, ice drift, and waves from space: the Sea surface KInematics Multiscale monitoring (SKIM) concept. Ocean Sci. 14(3): 337–354.
Aricò S., Sabine C., Wanninkhof R. 2021. Integrated ocean carbon research: a summary of ocean carbon research, and vision of coordinated ocean carbon research and observations for the next decade. p. 46.
Arrigo K.R., Van Dijken G., Pabi S. 2008. Impact of a shrinking Arctic ice cover on marine primary production. Geophys. Res. Lett. 35(19): 1–6.
Arrigo K.R., Perovich D.K., Pickart R.S., Brown Z.W., Van Dijken G.L., Lowry K.E., et al. 2012. Massive phytoplankton blooms under Arctic Sea ice. Science, 336(6087): 1408.
Årthun M., Eldevik T., Smedsrud L.H. 2019. The role of Atlantic heat transport in future Arctic winter sea ice loss. J. Clim. 32(11): 3327–3341.
Atamanchuk D., Koelling J., Send U., Wallace D.W.R. 2020. Rapid transfer of oxygen to the deep ocean mediated by bubbles. Nat. Geosci. 13(3): 232–237.
Atlas W., Housty W., Moore J. 2019. Indigenous community partnerships towards foundational understanding of wild Salmon survival. Technical Rep.(15): 69–70.
Aumont O., Maier-Reimer E., Blain S., Monfray P. 2003. An ecosystem model of the global ocean including Fe, Si, P colimitations. Global Biogeochem. Cycles, 17(2).
Azetsu-Scott K., Clarke A., Falkner K., Hamilton J., Jones E.P., Lee C., et al. 2010. Calcium carbonate saturation states in the waters of the Canadian Arctic Archipelago and the Labrador Sea. J. Geophys. Res.: Oceans, 115(11): 1–18.
Bach L.T., Gill S.J., Rickaby R.E.M., Gore S., Renforth P. 2019. CO2 removal with enhanced weathering and ocean alkalinity enhancement: potential risks and co-benefits for marine pelagic ecosystems. Front. Clim. 1(October).
Baker C.A., Martin A.P., Yool A., Popova E. 2022. Biological biological carbon pump sequestration efficiency in the North Atlantic: a leaky or a long-term sink? Global Biogeochem. Cycles, 36(6).
Bakker D.C.E., Pfeil B., Landa C.S., Metzl N., O'brien K.M., Olsen A., et al. 2016. A multi-decade record of high-quality fCO2 data in version 3 of the Surface Ocean CO2 Atlas (SOCAT). Earth Syst. Sci. Data, 8(2): 383–413.
Balaguru K., Doney S.C., Bianucci L., Rasch P.J., Leung L.R., Yoon J-Ho, Lima I.D. 2018. Linking deep convection and phytoplankton blooms in the northern Labrador Sea in a changing climate. PLoS One, 13(1): 1–17.
Ban N.C., Picard C.R., Vincent A.C.J. 2009. Comparing and integrating community-based and science-based approaches to prioritizing marine areas for protection. Conserv. Biol. 23(4): 899–910.
Ban N.C., Frid A., Reid M., Edgar B., Shaw D., Siwallace P. 2018. Incorporate Indigenous perspectives for impactful research and effective management. Nat. Ecol. Evol. 2(11): 1680–1683.
Barbière J., Isensee K., Schoo K. 2019. SDG 14.3.1 indicator methodology. Intergovernmental Oceanographic Commission of UNESCO. Available from https://www.iode.org/index.php?option=com_oe&task=viewDocumentRecord&docID=26155.
Barton A., Hales B., Waldbusser G.G., Langdon C., Feely R.A. 2012. The Pacific oyster, Crassostrea gigas, shows negative correlation to naturally elevated carbon dioxide levels: implications for near-term ocean acidification effects. Limnol. Oceanogr. 57(3): 698–710.
Bates N.R., Mathis J.T. 2009. The Arctic Ocean marine carbon cycle: evaluation of air–sea CO2 exchanges, ocean acidification impacts and potential feedbacks. Biogeosciences, 6(4): 2433–2459.
Becker J.M., Merrifield M.A., Yoon H. 2016. On the calculation of air–sea fluxes of CO2 in the presence of temperature and salinity gradients. J. Geophys. Res.: Oceans, 121(5): 3010–3028.
Bishop B., Oliver E.C.J., Aporta C. 2022. Co-producing maps as boundary objects: bridging Labrador Inuit knowledge and oceanographic research. J. Cult. Geogr. 39(1): 55–89.
Bond N.A., Cronin M.F., Freeland H., Mantua N. 2015. Causes and impacts of the 2014 warm anomaly in the NE Pacific. Geophys. Res. Lett. 42(9): 3414–3420.
Bourgeois T., Goris N., Schwinger J., Tjiputra J.F. 2022. Stratification constrains future heat and carbon uptake in the Southern Ocean between 30° S and 55° S. Nat. Commun. 13(1): 1–8.
Boyce J.K. 2018. Carbon pricing: effectiveness and equity. Ecol. Econ. 150: 52–61.
Boyd P.W., Claustre H., Levy M., Siegel D.A., Weber T. 2019. Multi-faceted particle pumps drive carbon sequestration in the ocean. Nature, 568, 327–335.
Bozhkov E., Walker C., Mccourt V., Castleden H. 2020. Are the natural sciences ready for truth, healing, and reconciliation with Indigenous peoples in Canada? Exploring “settler readiness” at a world-class freshwater research station. J. Environ. Stud. Sci. 10(3): 226–241.
Brady R.X., Lovenduski N.S., Alexander M.A., Jacox M., Gruber N. 2019. On the role of climate modes in modulating the air–sea CO2 fluxes in eastern boundary upwelling systems. Biogeosciences, 16(2): 329–346.
Breckwoldt A., Lopes P.F.M., Selim S.A. 2021. Look who's asking—reflections on participatory and transdisciplinary marine research approaches. Front. Mar. Sci. 8(June).
Briggs N., Perry M.J., Cetinić I., Lee C., D'asaro E., Gray A.M., Rehm E. 2011. High-resolution observations of aggregate flux during a sub-polar North Atlantic spring bloom. Deep-Sea Res., Part I, 58(10): 1031–1039.
Broecker W.S. 1979. A revised estimate for the radiocarbon age of North Atlantic deep water. J. Geophys. Res. 84(C6): 3218.
Bushinsky S.M., Landschützer P., Rödenbeck C., Gray A.R., Baker D., Mazloff M.R., et al. 2019. Reassessing southern ocean air–sea CO2 flux estimates with the addition of biogeochemical float observations. Global Biogeochem. Cycles, 33(11): 1370–1388.
Butterworth B.J., Else B.G.T. 2018. Dried, closed-path eddy covariance method for measuring carbon dioxide flux over sea ice. Atmos. Meas. Tech. 11: 6075–6090.
Capotondi A., Alexander M.A., Bond N.A., Curchitser E.N., Scott J.D. 2012. Enhanced upper ocean stratification with climate change in the CMIP3 models. J. Geophys. Res. 117: C04031.
Carley S., Konisky D.M. 2020. The justice and equity implications of the clean energy transition. Nat. Energy, 5(8): 569–577.
Chai F., Johnson K.S., Claustre H., Xing X., Wang Y., Boss E., et al. 2020. Monitoring ocean biogeochemistry with autonomous platforms. Nat. Rev. Earth Environ. 1(6): 315–326.
Chan F., Barth J.A., Blanchette C.A., Byrne R.H., Chavez F., Cheriton O., et al. 2017. Persistent spatial structuring of coastal ocean acidification in the California Current System. Sci. Rep. 7(1): 1–7.
Cheng W., Chiang J.C.H., Zhang D. 2013. Atlantic meridional overturning circulation (AMOC) in CMIP5 models: RCP and historical simulations. J. Clim. 26(18): 7187–7197.
Chierici M., Fransson A., Nojiri Y. 2006. Biogeochemical processes as drivers of surface fCO2 in contrasting provinces in the subarctic North Pacific Ocean. Global Biogeochem. Cycles, 20(1): 1–16.
Christensen J.P. 1994. Carbon export from continental shelves, denitrification and atmospheric carbon dioxide. Cont. Shelf Res. 14(5): 547–576.
Cooley S.R., Klinsky S., Morrow D.R., Satterfield T. 2022. Sociotechnical considerations about ocean carbon dioxide removal. Annu. Rev. Mar. Sci. 15, 1–26.
Cummins P.F., Ross T. 2020. Secular trends in water properties at Station P in the northeast Pacific : an updated analysis. Prog. Oceanogr. 186, 102329.
Dall'olmo G., Dingle J., Polimene L., Brewin R.J.W., Claustre H. 2016. Substantial energy input to the mesopelagic ecosystem from the seasonal mixed-layer pump. Nat. Geosci. 9(11): 820–823.
Davies H.N., Gould J., Hovey R.K., Radford B., Kendrick G.A. 2020. Mapping the marine environment through a cross-cultural collaboration. Front. Mar. Sci. 7(August): 1–15.
Degrandpre M., Evans W., Timmermans M.‐.L., Krishfield R., Williams B., Steele M. 2020. Changes in the Arctic Ocean carbon cycle with diminishing ice cover. Geophys. Res. Lett. 47(12): e2020GL088051.
Degrandpre M.D., Körtzinger A., Send U., Wallace D.W.R., Bellerby R.G.J. 2006. Uptake and sequestration of atmospheric CO2 in the Labrador Sea deep convection region. Geophys. Res. Lett. 33(21): 1–5.
Degrandpre M.D., Lai C‐Ze, Timmermans M.‐.L., Krishfield R.A., Proshutinsky A., Torres D. 2019. Inorganic carbon and pCO2 variability during ice formation in the Beaufort Gyre of the Canada basin. J. Geophys. Res.: Oceans, 124(6): 4017–4028.
Devries T. 2014. The oceanic anthropogenic CO2 sink: storage, air–sea fluxes, and transports over the industrial era. Global Biogeochem. Cycles, 28(7): 631–647.
deYoung B., Frajka-Williams E., von Oppeln-Bronikowski N., Woodward S. 2020. Technicalities: exploring the Labrador sea with autonomous vehicles. J. Ocean Technol. 15(3): 134–139.
Diggon S., Butler C., Heidt A., Bones J., Jones R., Outhet C. 2021. The marine plan partnership: Indigenous community-based marine spatial planning. Mar. Policy, 132(May): 103510.
Diggon S., Bones J., Short C.J., Smith J.L., Dickinson M., Wozniak K., et al. 2022. The Marine Plan Partnership for the North Pacific coast – MaPP: a collaborative and co-led marine planning process in British Columbia. Mar. Policy, 142: 104065.
Dinauer A., Mucci A. 2017. Spatial variability in surface-water pCO2 and gas exchange in the world's largest semi-enclosed estuarine system: St. Lawrence Estuary (Canada). Biogeosciences, 14(13): 3221–3237.
Dinauer A., Mucci A. 2018. Distinguishing between physical and biological controls on the spatial variability of pCO2: a novel approach using OMP water mass analysis (St. Lawrence, Canada). Mar. Chem. 204(April): 107–120.
Dion J., et al. 2021. Canada's net zero future: finding our way in the global transition. Canadian Institute for Climate Choices. Available from https://climateinstitute.ca/reports/canadas-net-zero-future/.
Doney S.C., Ruckelshaus M., Emmett Duffy J., Barry J.P., Chan F., English C.A., et al. 2012. Climate change impacts on marine ecosystems. Annu. Rev. Mar. Sci. 4: 11–37.
Doney S.C., Busch D.S., Cooley S.R., Kroeker K.J. 2020. The impacts of ocean acidification on marine ecosystems and reliant human communities. Ann. Rev. Environ. Resour. 45: 83–112.
Drever C.R., Cook-Patton S.C., Akhter F., Badiou P.H., Chmura G.L., Davidson S.J., et al. 2021. Natural climate solutions for Canada. Sci. Adv. 7(23): 1–14.
Dugdale R.C., Wilkerson F.P. 1991. Low specific nitrate uptake rate: a common feature of high-nutrient, low-chlorophyll marine ecosystems. Limnol. Oceanogr. 36(8): 1678–1688.
Duke P.J., Else B.G.T., Jones S.F., Marriot S., Ahmed M.M.M., Nandan V., et al. 2021. Seasonal marine carbon system processes in an arctic coastal landfast sea ice environment using an innovative underwater sensor platform. Elementa, 9(1): 1–21.
Durack P.J., Wijffels S.E., Matear R.J. 2012. Ocean salinities reveal strong global water cycle intensification during 1950 to 2000. Science, 336(April): 455–459.
Eger S.L., Stephenson R.L., Armitage D., Flannery W., Courtenay S.C. 2021. Revisiting integrated coastal and marine management in Canada: opportunities in the Bay of Fundy. Front. Mar. Sci. 8(May): 1–20.
Ekstrom J.A., Suatoni L., Cooley S.R., Pendleton L.H., Waldbusser G.G., Cinner J.E., et al. 2015. Vulnerability and adaptation of US shellfisheries to ocean acidification. Nat. Clim. Change, 5(3): 207–214.
Else B.G.T., Papakyriakou T.N., Galley R.J., Drennan W.M., Miller L.A., Thomas H. 2011. Wintertime CO2 fluxes in an Arctic polynya using eddy covariance: evidence for enhanced air-sea gas transfer during ice formation. J. Geophys. Res. 116(9): 1–15.
England M.H. 1995. The age of water and ventilation timescales in a global ocean model. J. Phys. Oceanogr. 25(11): 2756–2777.
Environment and Climate Change Canada. 2020. 2019 Canadian Carbon Cycle Research Workshop: report on key gaps and next steps. Available from https://publications.gc.ca/site/eng/9.893132/publication.html.
Evans W., Hales B., Strutton P.G., Ianson D. 2012. Sea-air CO2 fluxes in the western Canadian coastal ocean. Prog. Oceanogr. 101(1): 78–91.
Evans W., Mathis J.T. 2013. The Gulf of Alaska coastal ocean as an atmospheric CO2 sink. Cont. Shelf Res. 65: 52–63.
Evans W., Pocock K., Hare A., Weekes C., Hales B., Jackson J., et al. 2019. Marine CO2 patterns in the Northern Salish Sea. Front. Mar. Sci. 5: 1–18.
Evans W., Lebon G.T., Harrington C.D., Takeshita Y., Bidlack A. 2022. Marine CO2 system variability along the northeast Pacific Inside Passage determined from an Alaskan ferry. Biogeosciences, 19: 1277–1301.
Fabry V.J., Seibel B.A., Feely R.A., Orr J.C. 2008. Impacts of ocean acidification on marine biodiversity. ICES J. Mar. Sci. 65(3): 414–432.
Fassbender A.J., Rodgers K.B., Palevsky H.I., Sabine C.L. 2018a. Seasonal asymmetry in the evolution of surface ocean pCO2 and pH thermodynamic drivers and the influence on sea-air CO2 flux.Global Biogeochem. Cycles, 32(10): 1476–1497.
Fassbender A.J., Alin S.R., Feely R.A., Sutton A.J., Newton J.A., Krembs C., et al. 2018b. Seasonal carbonate chemistry variability in marine surface waters of the US Pacific Northwest. Earth Syst. Sci. Data, 10(3): 1367–1401.
Fay A.R., Mckinley G.A. 2013. Global trends in surface ocean pCO2 from in situ data. Global Biogeochem. Cycles, 27(August): 541–557.
Feely R.A., Sabine C.L., Hernandez-Ayon J.M., Ianson D., Hales B. 2008. Evidence for upwelling of corrosive “acidified” water onto the continental shelf. Science, 320(5882): 1490–1492.
Fennel K., Alin S., Barbero L., Evans W., Bourgeois T., Cooley S., et al. 2019. Carbon cycling in the North American coastal ocean: a synthesis. Biogeosciences, 16: 1281–1304.
Fisheries and Oceans Canada. 2019a. Aquaculture value added. Available from https://www.dfo-mpo.gc.ca/stats/aqua/va19pub-eng.htm [accessed 21 March 2022].
Fisheries and Oceans Canada. 2019b. Seafisheries value added. Available from https://www.dfo-mpo.gc.ca/stats/cfs-spc/tab/cfs-spc-tab1-eng.htm [accessed 21 March 2022].
Fong C., MacDougall S. 2023. Engineered carbon dioxide removal in a net-zero Canada: opportunities and challenges for non-biological CDR deployment. The Pembina Institute, Calgary, AB, Canada. Available from https://www.pembina.org/pub/engineered-carbon-dioxide-removal-net-zero-canada.
Ford D.J., Tilstone G.H., Shutler J.D., Kitidis V. 2022. Identifying the biological control of the annual and multi-year variations in South Atlantic air–sea CO2 flux. Biogeosciences, 19(17): 4287–4304.
Ford J.D., Smit B. 2004. A framework for the vulnerability of communities Arctic assessing to risks associated with climate change. Arctic, 57(4): 389–400.
Ford J.D., Berrang-Ford L., King M., Furgal C. 2010. Vulnerability of Aboriginal health systems in Canada to climate change. Global Environ. Change, 20(4): 668–680.
Ford J.D., Sherman M., Berrang-Ford L., Llanos A., Carcamo C., Harper S., et al. 2018. Preparing for the health impacts of climate change in Indigenous communities: the role of community-based adaptation. Global Environ. Change, 49(January): 129–139.
Franco A.C., Ianson D., Ross T., Hamme R.C., Monahan A.H., Christian J.R., et al. 2021. Anthropogenic and climatic contributions to observed carbon system trends in the Northeast Pacific. Global Biogeochem. Cycles, 1–21.
Freeland H. 2007. A short history of Ocean Station Papa and Line P. Prog. Oceanogr. 75: 120–125.
Freeland H.J., Crawford W.R., Thomson R.E. 1984. Currents along the pacific coast of canada. Atmos.-Ocean, 22(2): 151–172.
Freeland H.J. 2013. Evidence of change in the winter mixed layer in the Northeast Pacific Ocean : a problem revisited. Atmos.-Ocean, 51(1): 126–133.
Friedlingstein P., O'sullivan M., Jones M.W., Andrew R.M., Gregor L., Hauck J., et al. 2022. Global carbon budget 2022. Earth Syst. Sci. Data, 14(11): 4811–4900.
Friedrich T., Oschlies A. 2009. Neural network-based estimates of North Atlantic surface pCO2 from satellite data: A methodological study. J. Geophys. Res.: Oceans, 114(3): 1–12.
Frölicher T.L., Sarmiento J.L., Paynter D.J., Dunne J.P., Krasting J.P., Winton M. 2015. Dominance of the Southern Ocean in anthropogenic carbon and heat uptake in CMIP5 models. J. Clim. 28(2).
Frölicher T.L., Fischer E.M., Gruber N. 2018. Marine heatwaves under global warming. Nature, 560(7718): 360–364.
Gaudry A., Lorenz D. 2018. Indigenization as inclusion, reconciliation, and decolonization: navigating the different visions for indigenizing the Canadian Academy. AlterNative, 14(3): 218–227.
GESAMP. 2019. High level review of a wide range of proposed marine geoengineering techniques, GESAMP Reports and Studies. Joint Group of Experts on the Scientific Aspects of Marine Environmental Protections. p. 144. Available from www.imo.org.
Gloege L., Mckinley G.A., Landschützer P., Fay A.R., Frölicher T.L., Fyfe J.C., et al. 2021. Quantifying errors in observationally based estimates of ocean carbon sink variability. Global Biogeochem. Cycles, 35: e2020GB006788.
Godlewska A.M.C., Schaefli L.M., Forcione M., Lamb C., Nelson E., Talan B. 2020. Canadian colonialism, ignorance and education. A study of graduating students at Queen's University. J. Pedagogy, 11(1): 147–176.
Gooya P., Swart N.C., Hamme R.C. 2023. Time-varying changes and uncertainties in the CMIP6 ocean carbon sink from global to local scale. Earth Syst. Dyn. 14(2): 383–398.
Government of British Columbia. 2021. Roadmap to 2030. Government of British Columbia. Victoria, BC, Canada Available from https://communityclimatefunding.gov.bc.ca/the-cleanbc-roadmap-to-2030-has-been-released/.
Government of British Columbia. 2022. A coastal marine strategy for British Columbia: policy intentions paper. Government of British Columbia. Available from https://engage.gov.bc.ca/coastalmarinestrategy/ [accessed 6 April 2023].
Government of Canada. 2016. Pan-Canadian framework on clean growth and climate change : Canada's plan to address climate change and grow the economy. Government of Canada. Available from https://www.canada.ca/en/services/environment/weather/climatechange/pan-canadian-framework/climate-change-plan.html.
Government of Canada and Fisheries and Oceans Canada. 2018. A collaborative framework for joint DFO/NOAA ocean acidification research and monitoring. Available from https://www.dfo-mpo.gc.ca/science/publications/accasp-psaccma/noaa-collaborative/index-eng.html [accessed 3 April 2023].
Greenfield P. 2023. Revealed: more than 90% of rainforest carbon offsets by biggest certifier are worthless, analysis shows. The Guardian, 18 January. Available from https://www.theguardian.com/environment/2023/jan/18/revealed-forest-carbon-offsets-biggest-provider-worthless-verra-aoe [accessed 31 March 2023].
Gruber N., Clement D., Carter B.R., Feely R.A., Van Heuven S., Hoppema M., et al. 2019a. The oceanic sink for anthropogenic CO2 from 1994 to 2007. Science, 363(6432): 1193–1199.
Gruber N., Landschutzer P., Lovenduski N.S. 2019b. The variable southern ocean carbon sink. Annu. Rev. Mar. Sci. 11(159–86).
Haggan N., Jackson G.D., Lacroix P. 2009. Salmon and Eulachon in ecosystem space and time: a plea for wider collaboration and data integration. In Challenges for diadromous fishes in a dynamic global environment. Vol. 69. pp. 619–636.
Haigh R., Ianson D., Holt C.A., Neate H.E., Edwards A.M. 2015. Effects of ocean acidification on temperate coastal marine ecosystems and fisheries in the northeast pacific. PLoS One, 10(2): 1–46.
Hall A., Cox P., Huntingford C., Klein S. 2019. Progressing emergent constraints on future climate change. Nat. Clim. Change, 9(April): 269–278.
Hall M.M., Torres D.J., Yashayaev I. 2013. Absolute velocity along the AR7W section in the Labrador Sea. Deep-Sea Res., Part I, 72: 72–87.
Hansell D., Carlson C., Repeta D., Schlitzer R. 2009. Dissolved organic matter in the ocean: a controversy stimulates new insights. Oceanography, 22(4): 202–211.
Hansson L., Appeltans W., Gattuso J.-.P. 2014. Promoting international collaboration on ocean acidification data management. Trans., Am. Geophys. Union, 95(46): 421–422.
Hauri C., Pagès R., McDonnell A.M.P., Stuecker M.F., Danielson S.L., Hedstrom K., et al. 2021. Modulation of ocean acidification by decadal climate variability in the Gulf of Alaska. Commun. Earth Environ. 2(1): 1–7.
Ho D.T. 2023. Carbon dioxide removal is not a current climate solution—we need to change the narrative. Nature, 616(7955): 9.
Hunter K.L., Ross A.R.S., Ianson D., Miller L.A., Pearce C.M., Christian J.R., Pena M.A. 2015. Pacific Ocean Acidification Working Group –2014 /2015 report. Canadian Manuscript Report of Fisheries and Aquatic Sciences 3070. p. 23.
Hurd C.L., Law C.S., Bach L.T., Britton D., Hovenden M., Paine E.R., et al. 2022. Forensic carbon accounting: assessing the role of seaweeds for carbon sequestration. J. Phycol. 58(3): 347–363.
Ianson D., Allen S.E., Harris S.L., Orians K.J., Varela D.E., Wong C.S. 2003. The inorganic carbon system in the coastal upwelling region west of Vancouver Island, Canada. Deep-Sea Res., Part I, 50(8): 1023–1042.
Ianson D., Feely R.A., Sabine C.L., Juranek L.W. 2009. Features of coastal upwelling regions that determine net air–sea CO2 flux. J. Oceanogr. 65(5): 677–687.
Ianson D., Allen S.E. 2002. A two-dimensional nitrogen and carbon flux model in a coastal upwelling region. Global Biogeochem. Cycles, 16(1):.
Inuit Tapiriit Kanatami. 2018. National Inuit strategy on research: implementation plan. Available from https://www.canada.ca/en/research-coordinating-committee/priorities/indigenous-research/strategic-plan-2019-2022.html.
IPCC. 2013. IPCC, 2013: climate change 2013: the physical science basis. Contribution of Working Group I to the Fifth Assessment Report of the Intergovernmental Panel on Climate Change, Intergovernmental Panel of Climate Change. p. 1535.
IPCC. 2019. Chapter 5: changing ocean, marine ecosystems, and dependent communities. Intergovernmental Panel of Climate Change Special Report for the Ocean and Cryosphere in the Changing Climate. pp. 1–198.
IPCC. 2023. AR6 synthesis report: climate change 2023—IPCC. Cambridge University Press, Cambridge, UK: Available from https://www.ipcc.ch/report/sixth-assessment-report-cycle/ [accessed 31 March 2023].
Izett R.W., Castro De La Guardia L., Chanona M., Myers P.G., Waterman S., Tortell P.D. 2022. Impact of vertical mixing on summertime net community production in Canadian Arctic and Subarctic waters: insights from in situ measurements and numerical simulations. J. Geophys. Res.: Oceans, 127(8): 1–26.
Jarníková T., Ianson D., Allen S.E., Shao A.E., Olson E.M. 2022. Anthropogenic carbon increase has caused critical shifts in aragonite saturation across a sensitive coastal system. Global Biogeochem. Cycles. In press.
Jones D.C., Ito T., Takano Y., Hsu W.-.C. 2014. Spatial and seasonal variability of the air–sea equilibration timescale of carbon dioxide. Global Biogeochem. Cycles, 28: 1163–1178.
Kater I. 2022. Natural and Indigenous sciences: reflections on an attempt to collaborate. Region. Environ. Change, 22(4): 109.
Kenny T.-.A., Archambault P., Ayotte P., Batal M., Chan H.M., Cheung W., et al. 2020. Oceans and human health—navigating changes on Canada's coasts. Facets, 5(1): 1037–1070.
Khatiwala S., Tanhua T., Mikaloff Fletcher S., Gerber M., Doney S.C., Graven H.D., et al. 2013. Global ocean storage of anthropogenic carbon. Biogeosciences, 10(4): 2169–2191.
Körtzinger A., Send U., Wallace D.W.R., Karstensen J., Degrandpre M. 2008. Seasonal cycle of O2 and pCO2 in the central Labrador Sea: atmospheric, biological, and physical implications. Global Biogeochem. Cycles, 22(1): 1–16.
Kovach M. 2021. Indigenous methodologies: characteristics, conversations, and contexts. 2nd ed. University of Toronto Press.
Lacour L., Briggs N., Claustre H., Ardyna M., Dall'olmo G. 2019. The intraseasonal dynamics of the mixed layer pump in the subpolar North Atlantic Ocean: a Biogeochemical-Argo float approach. Global Biogeochem. Cycles, 33(3): 266–281.
Laframboise S.J., Bailey T., Dang A.-.T., Rose M., Zhou Z., Berg M.D., et al. 2023. Analysis of financial challenges faced by graduate students in Canada. Biochem. Cell Biol. In press.
Landschützer P., Gruber N., Bakker D.C.E., Schuster U. 2014. Recent variability of the global ocean carbon sink. Global Biogeochem. Cycles, 28(9): 927–949.
Landschützer P., Gruber N., Bakker D.C.E., Stemmler I., Six K.D. 2018. Strengthening seasonal marine CO2 variations due to increasing atmospheric CO2. Nat. Clim. Change, 8(2): 146–150.
Lannuzel D., Tedesco L., Van Leeuwe M., Campbell K., Flores H., Delille B., et al. 2020. The future of Arctic sea-ice biogeochemistry and ice-associated ecosystems. Nat. Clim. Change, 10(11): 983–992.
Laruelle G.G., Lauerwald R., Pfeil B., Regnier P. 2014. Regionalized global budget of the CO2 exchange at the air-water interface in continental shelf seas. Global Biogeochem. Cycles, 28: 1199–1214.
Laruelle G.G., Cai W.-.J., Hu X., Gruber N., Mackenzie F.T., Regnier P. 2018. Continental shelves as a variable but increasing global sink for atmospheric carbon dioxide. Nat. Commun. 9(454): 1–11.
Laxon S.W., Giles K.A., Ridout A.L., Wingham D.J., Willatt R., Cullen R., et al. 2013. CryoSat-2 estimates of Arctic sea ice thickness and volume. Geophys. Res. Lett. 40(4): 732–737.
Legge O., Johnson M., Hicks N., Jickells T., Diesing M., Aldridge J., et al. 2020. Carbon on the Northwest European Shelf: contemporary budget and future influences. Front. Mar. Sci. 7(March).
Lezaun J. 2021. Hugging the shore: tackling marine carbon dioxide removal as a local governance problem. Front. Clim. 3(August): 1–6.
Li F., Lozier M.S. 2018. On the linkage between Labrador Sea Water volume and overturning circulation in the Labrador Sea: a case study on proxies. J. Clim. 31(13): 5225–5241.
Li H., Ilyina T., Müller W.A., Landschützer P. 2019. Predicting the variable ocean carbon sink. Sci. Adv. 5(4): 1–9.
Lindoso V. 2019. Choices made now are critical for the future of our ocean and cryosphere, IOC-UNESCO. Available from https://en.unesco.org/news/choices-made-now-are-critical-future-our-ocean-and-cryosphere [accessed 19 March 2022].
Lombard A.T., Ban N.C., Smith J.L., Lester S.E., Sink K.J., Wood S.A., et al. 2019. Practical approaches and advances in spatial tools to achieve multi-objective marine spatial planning. Front. Mar. Sci. 6: 1–9.
Loomis R., Cooley S.R., Collins J.R., Engler S., Suatoni L. 2022. A code of conduct is imperative for ocean carbon dioxide removal research. Front. Mar. Sci. 9. Available from https://www.frontiersin.org/articles/10.3389/fmars.2022.872800 [accessed 28 March 2023].
Maldonado J.K., Shearer C., Bronen R., Peterson K., Lazrus H. 2013. The impact of climate change on tribal communities in the US: displacement, relocation, and human rights. Clim. Change, 120(3): 601–614.
Manning C.C., Preston V.L., Jones S.F., Michel A.P.M., Nicholson D.P., Duke P.J., et al. 2020. River inflow dominates methane emissions in an Arctic coastal system. Geophys. Res. Lett. 47: e2020GL087669.
Marine Planning Partnership Initiative. 2015a. Central coast marine plan. p. 24.
Marine Planning Partnership Initiative. 2015b. Haida Gwaii marine plan.
Marine Planning Partnership Initiative. 2015c. North coast marine plan. p. 166.
Marine Planning Partnership Initiative. 2015d. North Vancouver Island marine plan. p. 163.
Marshall J., Dobson F., Moore K., Rhines P., Visbeck M., d'Asaro E., et al. 1998. The Labrador Sea deep convection experiment. Bull. Am. Meteorol. Soc. 79(10): 2033–2058.
Marshall J., Kushnir Y., Battisti D., Chang P., Czaja A., Dickson R., et al. 2001. North Atlantic climate variability: phenomena, impacts and mechanisms. Int. J. Climatol. 21(15): 1863–1898.
Martin J.H., Coale K.H., Johnson K.S., Fitzwater S.E., Gordon R.M., Tanner S.J., et al. 1994. Testing the iron hypothesis in ecosystems of the equatorial Pacific Ocean. Nature, 371(September): 123–129.
Martz T.R., Degrandpre M.D., Strutton P.G., Mcgillis W.R., Drennan W.M. 2009. Sea surface pCO2 and carbon export during the Labrador Sea spring-summer bloom: an in situ mass balance approach. J. Geophys. Res.: Oceans, 114(9): 1–12.
Maru Y.T., Stafford Smith M., Sparrow A., Pinho P.F., Dube O.P. 2014. A linked vulnerability and resilience framework for adaptation pathways in remote disadvantaged communities. Global Environ. Change, 28, 337–350.
Maslanik J.A., Fowler C., Stroeve J., Drobot S., Zwally J., Yi D., Emery W. 2007. A younger, thinner Arctic ice cover: increased potential for rapid, extensive sea-ice loss. Geophys. Res. Lett. 34(24).
Matthes L.C., Ehn J.K., Dalman L.A., Babb D.G., Peeken I., Harasyn M., et al. 2021. Environmental drivers of spring primary production in Hudson Bay. Elementa, 9(1): 00160.
Mayor R. 2021. Removing forward: centering equity and justice in a carbon-removing future. Carbon180.
McGregor D. 2018. From “decolonized” to reconciliation research in Canada: drawing from indigenous research paradigms. ACME, 17(3): 810–831.
Mckinley G.A., Fay A.R., Takahashi T., Metzl N. 2011. Convergence of atmospheric and North Atlantic carbon dioxide trends on multidecadal timescales. Nat. Geosci. 4(July).
Mckinley G.A., Pilcher D.J., Fay A.R., Lindsay K., Long M.C., Lovenduski N.S. 2016. Timescales for detection of trends in the ocean carbon sink. Nature, 530(7591): 469–472.
Mckinley G.A., Fay A.R., Eddebbar Y.A., Gloege L., Lovenduski N.S. 2020. External forcing explains recent decadal variability of the ocean carbon sink. AGU Adv. 1(2): 1–10.
Mclean E.L., Katenka N.V., Seibel B.A. 2018. Decreased growth and increased shell disease in early benthic phase Homarus americanus in response to elevated CO2. Mar. Ecol. Prog. Series, 596: 113–126.
Messié M., Chavez F.P. 2015. Seasonal regulation of primary production in eastern boundary upwelling systems. Prog. Oceanogr. 134: 1–18.
Mogen S.C., Lovenduski N.S., Dallmann A.R., Gregor L., Sutton A.J., Bograd S.J., et al. 2022. Ocean biogeochemical signatures of the North Pacific Blob. Earth Space Sci. Open Archive, 49: e2021GL096938.
Moore S.E., Grebmeier J.M. 2018. The distributed biological observatory: linking physics to biology in the Pacific Arctic Region. Arctic, 71: 1–7.
Moran K., Boutin B., Juniper S.K., Pirenne B., Round A. 2022. A multi-use and multi-stakeholder ocean observing platform system, OCEANS 2019 MTS/IEEE Seattle, 0–4.
Mortenson E., Steiner N., Monahan A.H., Hayashida H., Sou T., Shao A. 2020. Modeled impacts of sea ice exchange processes on Arctic Ocean carbon uptake and acidification (1980–2015). J. Geophys. Res.: Oceans, 125(7): 1–20.
NASEM. 2021. A research strategy for ocean-based carbon dioxide removal and sequestration. National Academies of Sciences, Engineering, and Medicine, Washington, DC.
Nemcek N., Ianson D., Tortell P.D. 2008. A high-resolution survey of DMS, CO2, and O2/Ar distributions in productive coastal waters. Global Biogeochem. Cycles, 22(2): 1–13.
Newton J.A., Feely R.A., Jewett E.B., Williamson P., Mathis J. 2015. Global ocean acidification observing network: requirements and governance plan. 57p.
Nishino S., Itoh M., Kawaguchi Y., Kikuchi T., Aoyama M. 2011. Impact of an unusually large warm-core eddy on distributions of nutrients and phytoplankton in the southwestern Canada Basin during late summer/early fall 2010. Geophys. Res. Lett. 38(16): 1–6.
O'neill B.C., Tebaldi C., Van Vuuren D.P., Eyring V., Friedlingstein P., Hurtt G., et al. 2018. The Scenario Model Intercomparison Project (ScenarioMIP) for CMIP6. pp. 3461–3482.
Orr J.C., Fabry V.J., Aumont O., Bopp L., Doney S.C., Feely R.A., et al. 2005. Anthropogenic ocean acidification over the twenty-first century and its impact on calcifying organisms. Nature, 437(7059): 681–686.
Orr J.C., Epitalon J.-.M., Dickson A.G., Gattuso J.-.P. 2018. Routine uncertainty propagation for the marine carbon dioxide system. Mar. Chem. 207(October): 84–107.
Osiecka A.N., Wróbel A., Hendricks I.-.W., Osiecka-Brzeska K. 2022. Being ERC in marine science: results of a survey among early-career marine scientists and conservationists. Front. Mar. Sci. 9: 835692.
Oubanas H., Gejadze I., Malaterre P.‐.O., Durand M., Wei R., Frasson R.P.M., Domeneghetti A. 2018. Discharge estimation in ungauged basins through variational data assimilation: the potential of the SWOT mission. Water Resour. Res. 54(3): 2405–2423.
Paasche Ø., Olsen A., Årthun M., Anderson L.G., Wängberg S.-Å., Ashjian C.J., et al. 2019. Addressing Arctic challenges requires a synoptic ocean survey, Eos. Available from http://eos.org/opinions/addressing-arctic-challenges-requires-a-synoptic-ocean-survey [accessed 7 April 2023].
Palevsky H.I., Ribalet F., Swalwell J.E., Cosca C.E., Cokelet E.D., Feely R.A., et al. 2013. The influence of net community production and phytoplankton community structure on CO2 uptake in the Gulf of Alaska. Global Biogeochem. Cycles.
Pedersen C., Otokiak M., Koonoo I., Milton J., Maktar E., Anaviapik A., et al. 2020. ScIQ: an invitation and recommendations to combine science and Inuit Qaujimajatuqangit for meaningful engagement of inuit communities in research. Arct. Sci. 6(3): 326–339.
Peng W. 2020. Trade-offs for equitable climate policy assessed. Nature, 588(7837): 225–226.
Perovich D.K., Light B., Eicken H., Jones K.F., Runciman K., Nghiem S.V. 2007. Increasing solar heating of the Arctic Ocean and adjacent seas, 1979-2005: attribution and role in the ice-albedo feedback. Geophys. Res. Lett. 34(19).
Peters G.P., Le Quéré C., Andrew R.M., Canadell J.G., Friedlingstein P., Ilyina T., et al. 2017. Towards real-time verification of CO2 emissions. Nat. Clim. Change, 7(12): 848–850.
Pörtner H.O., et al. 2019. IPCC special report on the ocean and cryosphere in a changing climate. IPCC Intergovernmental Panel on Climate Change, Geneva, Switzerland. Available from https://www.ipcc.ch/srocc/ [accessed 31 March 2023].
Proulx M., Ross L., Macdonald C., Fitzsimmons S., Smit M. 2021. Indigenous traditional ecological knowledge and ocean observing: a review of successful partnerships. Front. Mar. Sci. 8(July): 1–18.
Prowse T., Bring A., Mård J., Carmack E., Holland M., Instanes A., et al. 2015. Arctic freshwater synthesis: summary of key emerging issues. J. Geophys. Res.: Biogeosci. 120(10): 1887–1893.
Rainville L., Lee C., Woodgate R. 2011. Sea ice biogeochemistry and material transport across the frozen interface. Oceanography, 24(3): 202–218.
Reid A.J., Eckert L.E., Lane J.‐.F., Young N., Hinch S.G., Darimont C.T., et al. 2021. “Two-Eyed Seeing”: an Indigenous framework to transform fisheries research and management. Fish Fish. 22(2): 243–261.
Resplandy L., Séférian R., Bopp L. 2015. Natural variability of CO2 and O2 fluxes: what can we learn from centuries-long climate models simulations?. J. Geophys. Res.: Oceans, 120(1): 384–404.
Resplandy L., Lévy M., Mcgillicuddy D.J. 2019. Effects of eddy-driven subduction on ocean biological carbon pump. Global Biogeochem. Cycles, 33(8): 1071–1084.
Ribalet F., Marchetti A., Hubbard K.A., Brown K., Durkin C.A., Morales R., et al. 2010. Unveiling a phytoplankton hotspot at a narrow boundary between coastal and offshore waters. Proc. Natl. Acad. Sci. U.S.A. 107(38): 16571–16576.
Rieck J.K., Böning C.W., Getzlaff K. 2019. The nature of eddy kinetic energy in the labrador sea: different types of mesoscale eddies, their temporal variability, and impact on deep convection. J. Phys. Oceanogr. 49(8): 2075–2094.
Ringuette M., Devred E., Azetsu-Scott K., Head E., Punshon S., Casault B., Clay S. 2022. Optical, chemical, and biological oceanographic conditions in the Labrador Sea between 2014 and 2018. DFO Can. Sci. Advis. Sec. Res. Doc.
Rivers N., Strand M., Fernandes M., Metuge D., Lemahieu A., Nonyane C.L., et al. 2023. Pathways to integrate Indigenous and local knowledge in ocean governance processes: lessons from the Algoa Bay Project. Front. Mar. Sci. 9(1084674).
Ross T., Hannah C., Whitney F. 2019. The Northeast Pacific: current status and recent trends. PICES Press, 27(1): 36–39.
Roth F., Broman E., Sun X., Bonaglia S., Nascimento F., Prytherch J., et al. 2023. Methane emissions offset atmospheric carbon dioxide uptake in coastal macroalgae, mixed vegetation and sediment ecosystems. Nat. Commun. 14(1): 42.
Rutherford K., Fennel K., Atamanchuk D., Wallace D., Thomas H. 2021. A modelling study of temporal and spatial pCO2 variability on the biologically active and temperature-dominated Scotian Shelf. Biogeosciences, 18(23): 6271–6286.
Rysgaard S., Nielsen T.G, Hansen B.W. 1999. Seasonal variation in nutrients, pelagic primary production and grazing in a high-Arctic coastal marine ecosystem, Young Sound, Northeast Greenland.Mar. Ecol. Prog. Series, 179(Digby 1953): 13–25.
Rysgaard S., Glud R.N., Sejr M.K., Bendtsen J., Christensen P.B. 2007. Inorganic carbon transport during sea ice growth and decay: a carbon pump in polar seas. J. Geophys. Res. 112(3): 1–8.
Rysgaard S., Bendtsen J., Delille B., Dieckmann G.S., Glud R.N., Kennedy H., et al. 2011. Sea ice contribution to the air–sea CO2 exchange in the Arctic and Southern Oceans. Tellus, Series B: Chem. Phys. Meteorol. 63(5): 823–830.
Rysgaard S., Søgaard D.H., Cooper M., Pućko M., Lennert K., Papakyriakou T.N., et al. 2013. Ikaite crystal distribution in winter sea ice and implications for CO2 system dynamics. Cryosphere, 7(2): 707–718.
Sarmiento J.L., Hughes T.M.C., Stouffer R.J., Manabe S. 1998. Simulated response of the ocean carbon cycle to anthropogenic climate warming. Nature, 393(6682): 245–249.
Sastri A.R., Christian J.R., Achterberg E.P., Atamanchuk D., Buck J.J.H., Bresnahan P., et al. 2019. Perspectives on in situ Sensors for Ocean Acidification Research. Front. Mar. Sci. 6: 653.
Shadwick E.H., Thomas H., Azetsu-Scott K., Greenan B.J.W., Head E., Horne E. 2011. Seasonal variability of dissolved inorganic carbon and surface water pCO2 in the Scotian Shelf region of the Northwestern Atlantic. Mar. Chem. 124(1–4): 23–37.
Shutler J.D. 2020. Offsetting is a dangerous smokescreen for inaction. Front. Ecol. Environ. 18(9): 486.
Shutler J.D., Wanninkhof R., Nightingale P.D., Woolf D.K., Bakker D.C.E, Watson A., et al. 2020. Satellites will address critical science priorities for quantifying ocean carbon. Front. Ecol. Environ. 18(1): 27–35.
Signorini S.R., Mannino A., Najjar R.G., Friedrichs M.A.M., Cai W.-.J., Salisbury J., et al. 2013. Surface ocean pCO2 seasonality and sea-air CO2 flux estimates for the North American east coast. J. Geophys. Res.: Oceans, 118(10): 5439–5460.
Sonnichsen C., Atamanchuk D., Hendricks A., Morgan S., Smith J., Grundke I., et al. 2023. An automated microfluidic analyzer for in situ monitoring of total alkalinity. ACS Sensors, 8(1): 344–352.
Søreide J.E., Leu E., Berge J., Graeve M., Falk-Petersen S. 2010. Timing of blooms, algal food quality and Calanus glacialis reproduction and growth in a changing Arctic. Global Change Biol. 16(11): 3154–3163.
Sothe C., Gonsamo A., Arabian J., Kurz W.A., Finkelstein S.A., Snider J. 2022. Large soil carbon storage in terrestrial ecosystems of Canada. Global Biogeochem. Cycles, 36(2): 1–18.
Stewart A., Deyoung B., Smit M., Donaldson K., Reedman A., Bastien A., et al. 2019. The development of a Canadian Integrated Ocean Observing System (CIOOS). Front. Mar. Sci. 6. Available from https://www.frontiersin.org/articles/10.3389/fmars.2019.00431 [accessed 23 March 2023].
Sutton A.J., Wanninkhof R., Sabine C.L., Feely R.A., Cronin M.F., Weller R.A. 2017. Variability and trends in surface seawater pCO2 and CO2 flux in the Pacific Ocean. Geophys. Res. Lett. 44(11): 5627–5636.
Sutton A.J., Feely R.A., Maenner-Jones S., Musielwicz S., Osborne J., Dietrich C., et al. 2019. Autonomous seawater pCO2 and pH time series from 40 surface buoys and the emergence of anthropogenic trends. Earth Syst. Sci. Data, 11(1): 421–439.
Tanhua T., Pouliquen S., Hausman J., O'brien K., Bricher P., De Bruin T., et al. 2019. Ocean FAIR data services. Front. Mar. Sci. 6. Available from https://www.frontiersin.org/articles/10.3389/fmars.2019.00440 [accessed 19 April 2023].
Therriault J.-.C., Petrie B., Pepin P., Gagnon J., Gregory D., Helbig J. 1998. Proposal for a northwest Atlantic zonal monitoring program. Can. Tech. Rep. Hydrogr. Ocean Sci. 194: vii+57.
Thomas H., Friederike Prowe A.E., Lima I.D., Doney S.C., Wanninkhof R., Greatbatch R.J., et al. 2008. Changes in the North Atlantic oscillation influence CO2 uptake in the North Atlantic over the past 2 decades. Global Biogeochem. Cycles, 22(4): 1–13.
Timmermans M.‐.L., Marshall J. 2020. Understanding Arctic Ocean circulation: a review of ocean dynamics in a changing climate. J. Geophys. Res.: Oceans, 125(4): 1–35.
Tremblay J.-.É., Anderson L.G., Matrai P., Coupel P., Bélanger S., Michel C., Reigstad M. 2015. Global and regional drivers of nutrient supply, primary production and CO2 drawdown in the changing Arctic Ocean. Prog. Oceanogr. 139: 171–196.
Truth and Reconciliation Commission of Canada. 2015. Truth and Reconciliation Commission of Canada: calls to action, calls to action. Winnipeg, Manitoba, Canada. p. 20. Available from https://nctr.ca/records/reports/#trc-reports.
Turgeon K., Hawkshaw S.C.F., Dinning K.M., Quinn B.K., Edwards D.N., Wor C., et al. 2018. Enhancing fisheries education and research through the Canadian Fisheries Research Network: a student perspective on interdisciplinarity, collaboration and inclusivity. Facets, 3(1): 963–980.
Ullman D.J., Mckinley G.A., Bennington V., Dutkiewicz S. 2009. Trends in the North Atlantic carbon sink: 1992–2006.Global Biogeochem. Cycles, 23(4): 1992–2006.
Verra. 2022. Verified Carbon Standard Program Guide v4.1. (January). Available from https://verra.org/programs/verified-carbon-standard/vcs-version-4-rules-and-requirements/.
Villa-Alfageme M., De Soto F.C., Ceballos E., Giering S.L.C., Le Moigne F.A.C., et al. 2016. Geographical, seasonal, and depth variation in sinking particle speeds in the North Atlantic. Geophys. Res. Lett. 43: 8609–8616.
Vonk J.E., Gustafsson Ö. 2013. Permafrost-carbon complexities. Nat. Geosci. 6(9): 675–676.
Wang H., Hu X., Sterba-Boatwright B. 2016. A new statistical approach for interpreting oceanic fCO2 data. Mar. Chem. 183: 41–49.
Wang Q., Wekerle C., Danilov S., Koldunov N., Sidorenko D., Sein D., et al. 2018. Arctic Sea ice decline significantly contributed to the unprecedented liquid freshwater accumulation in the Beaufort Gyre of the Arctic Ocean. Geophys. Res. Lett. 45(10): 4956–4964.
Wanninkhof R., Asher W.E., Ho D.T., Sweeney C., Mcgillis W.R. 2009. Advances in quantifying air–sea gas exchange and environmental forcing. Ann. Rev. Mar. Sci. 1: 213–244.
Wanninkhof R., Park G.-.H., Takahashi T., Sweeney C., Feely R., Nojiri Y., et al. 2013. Global ocean carbon uptake : magnitude, variability and trends. Biogeosciences, 10: 1983–2000.
Watson A.J., Schuster U., Shutler J.D., Holding T., Ashton I.G.C., Landschützer P., et al. 2020. Revised estimates of ocean–atmosphere CO2 flux are consistent with ocean carbon inventory. Nat. Commun. 11(1): 1–6.
Weatherdon L.V., Ota Y., Jones M.C., Close D.A., Cheung W.W.L. 2016. Projected scenarios for coastal first nations’ fisheries catch potential under climate change: management challenges and opportunities. PLoS One, 11(1): 1–28.
Weiss K., Hamann M., Marsh H. 2013. Bridging knowledges: understanding and applying Indigenous and Western scientific knowledge for marine wildlife management. Soc. Nat. Resour. 26(3): 285–302.
Westbrook G.K., Thatcher K.E., Rohling E.J., Piotrowski A.M., Pälike H., Osborne A.H., et al. 2009. Escape of methane gas from the seabed along the West Spitsbergen continental margin. Geophys. Res. Lett. 36(15): 1–5.
Williamson P., Gattuso J.-P. 2022. Carbon removal using coastal blue carbon ecosystems is uncertain and unreliable, with questionable climatic cost-effectiveness. Front. Clim. 4(853666): 1–14.
Wilson K., Arreak A., Committee S., Bell T., Ljubicic G. 2021. The Mittimatalik Siku Asijjipallianinga (Sea Ice Climate Atlas): how Inuit knowledge, earth observations, and sea ice charts can fill IPCC climate knowledge gaps. Front. Clim. 3. Available from https://www.frontiersin.org/articles/10.3389/fclim.2021.715105 [accessed: 28 March 2023].
Winton M., Griffies S.M., Samuels B.L., Sarmiento J.L., Frölicher T.L. 2013. Connecting changing ocean circulation with changing climate. J. Clim. 26(7): 2268–2278.
Wong C., Ballegooyen K., Ignace L., Johnson M.J.(G.), Swanson H. 2020. Towards reconciliation: 10 calls to action to natural scientists working in Canada. Facets, 5(1): 769–783.
Wong C.S., Chan Y.-H. 1991. Temporal variations in the partial pressure and flux of CO2 at ocean station P in the subarctic northeast Pacific Ocean. Tellus, 43B: 206–223.
Wong C.S., Christian J.R., Emmy Wong S.-K., Page J., Xie L., Johannessen S. 2010. Carbon dioxide in surface seawater of the eastern North Pacific Ocean (Line P), 1973–2005. Deep-Sea Res., Part I, 57(5): 687–695.
Wunsch C. 2002. What Is the predictive ecology. Science, 298(November): 1179–1180.
Yamamoto‐Kawai M., Mclaughlin F.A., Carmack E.C., Nishino S., Shimada K., Kurita N. 2009. Surface freshening of the Canada Basin, 2003–2007: river runoff versus sea ice meltwater. J. Geophys. Res. 114(C1): C00A05.
Yashayaev I., Loder J.W. 2017. Further intensification of deep convection in the Labrador Sea in 2016. Geophys. Res. Lett. 44(3): 1429–1438.
Supplementary material
Supplementary Material 1 (DOCX / 150 KB).
- Download
- 150.87 KB
Information & Authors
Information
Published In
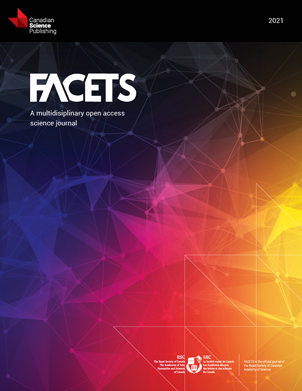
FACETS
Volume 8 • 2023
Pages: 1 - 21
Editor: Dawn Kellett
History
Received: 4 October 2022
Accepted: 2 May 2023
Version of record online: 20 July 2023
Notes
This paper is part of a collection entitled "Resources for Early Career Researchers: A Collection of Guides & Perspectives".
Copyright
© 2023 The Author(s). This work is licensed under a Creative Commons Attribution 4.0 International License (CC BY 4.0), which permits unrestricted use, distribution, and reproduction in any medium, provided the original author(s) and source are credited.
Data Availability Statement
Data analyzed during this study are provided in full within supplementary materials Table S1.
Key Words
Sections
Subjects
Authors
Author Contributions
Conceptualization: PJD, BR, RA, JL, KS, PG, MMMA, MRM, CAB, KK, RP, YS, GN, ACF
Data curation: PJD, BR, RA, ACF
Formal analysis: PJD, RA, KK, ACF
Investigation: PJD, BR, RA, JL, KS, PG, MMMA, MRM, CAB, KK, RP, YS, GN
Project administration: PJD
Validation: ACF
Visualization: BR
Writing – original draft: PJD, BR, RA, JL, KS, PG, MMMA, MRM, CAB, KK, RP, YS, GN, ACF
Writing – review & editing: PJD, BR, RA, JL, KS, PG, MMMA, MRM, CAB, KK, RP, YS, GN, ACF
Competing Interests
The authors declare that they have no conflicts of interest.
Funding Information
Funding for this project was provided by the Natural Sciences and Engineering Research Council of Canada (NSERC) through the Advancing Climate Change Science in Canada program (grant # ACCPJ 536173-18) to Roberta Hamme.
Metrics & Citations
Metrics
Other Metrics
Citations
Cite As
P.J. Duke, B. Richaud, R. Arruda, J. Länger, K. Schuler, P. Gooya, M.M.M. Ahmed, M.R. Miller, C.A. Braybrook, K. Kam, R. Piunno, Y. Sezginer, G. Nickoloff, and A.C. Franco. 2023. Canada's marine carbon sink: an early career perspective on the state of research and existing knowledge gaps. FACETS.
8: 1-21.
https://doi.org/10.1139/facets-2022-0214
Export Citations
If you have the appropriate software installed, you can download article citation data to the citation manager of your choice. Simply select your manager software from the list below and click Download.
Cited by
1. Global priorities for ocean sustainability from Early Career Ocean Professionals
2. Feedback Mechanisms Between Atmospheric and Oceanic Factors on Atmospheric CO2 Concentration Over Tropical Coastal Waters
3. Seasonality of pCO2 and air-sea CO2 fluxes in the Central Labrador Sea
4. Assessment and Spatio-Temporal Evolution of Marine Fisheries’ Carbon Sink Capacity in China’s Three Marine Economic Circles
5. Arctic Oceanic Carbon Cycle: A Comprehensive Review of Mechanisms, Regulations, and Models
6. Estimating marine carbon uptake in the northeast Pacific using a neural network approach